
5 minute read
DRAINAGE USING GEOCOMPOSITES
from IMIESA July 2023
by 3S Media
The movement of groundwater is facilitated by the presence of voids or pores in the ground. These voids allow water to rise to the surface, particularly in areas with significant changes in relative levels such as steep slopes, intersections of rock and soil masses, or boundaries between soil masses with varying permeability.
By Janita Ramanlal*
Advertisement
However, when man-made structures impede this natural flow, it becomes essential to implement specific measures to preserve the integrity of the completed structure.
Traditionally, subsoil drains composed of sand and gravel have been employed to divert groundwater away from structures, ensuring their integrity throughout their intended design life. Drainage geocomposites offer a viable alternative to these conventional drains (see Figure 1).
Utilising drainage geocomposites presents numerous advantages, with the most notable being the reduction in construction costs and time. Minimising quarrying, and transportation of large quantities of natural materials to the site, reduces overall costs and installation duration. Furthermore, drainage geocomposites are lightweight and manageable, requiring less time and manpower to install than traditional solutions.
Compared to conventional gravel drains, drainage geocomposites can provide up to three times the drainage capacity with 60% less excavation. This makes them particularly advantageous in scenarios where the mineral layer of steep slopes would be unstable, as drainage geocomposites offer stability without compromising drainage efficiency. Under practical usage conditions, the drainage capacity of these geocomposites is measured in laboratories, and their performance is guaranteed by quality control.
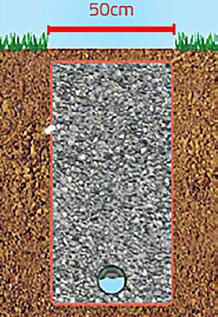
Drainage core
Drainage geocomposites are composed of a drainage core (Figure 2) that enables efficient transportation of fluid along its plane. To prevent clogging of the core by surrounding soil, geotextiles and/or geomembranes are coupled to the drainage core. The most widely employed drainage geocomposites are those produced by laminating one or two geotextiles with a filtering function onto a drainage element.
The geotextiles (typically non-woven) serving as the filtering component must have adequate permeability to gases and liquids in the direction perpendicular to the filter plane and must be able to separate the soil particles from the drainage core. The drainage core, which serves as the draining component, must have adequate permeability to gases and liquids in the direction planar to the drainage structure. When designed correctly, drainage geocomposites provide an effective solution for fluid management.
Typical applications
Some of the main applications of drainage geocomposites are (and are not limited to):
• Retaining structures: Drainage geocomposites dissipate pore water pressures and help maintain the stability and integrity of the structural fill in both concrete and soilreinforced retaining structures.
• Road, railway, and airport applications: Wick/ trench drains, which can substitute subsoil drains, are commonly used in road, railway and airport projects. Drainage geocomposites are utilised in these applications to provide efficient water drainage, ensuring optimal performance and durability.
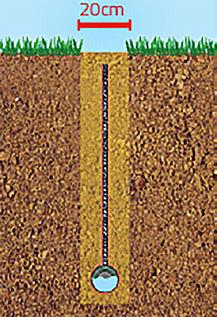
• Horizontal drainage and anti-capillary layers in embankments composed of fine and cohesive fills: Drainage geocomposites facilitate the removal of excess water from the embankment, preventing water accumulation and potential instability. Additionally, they can act as anti-capillary layers, preventing the upward movement of water through the embankment.
• Landfills: Drainage geocomposites are utilised for leachate collection and gas ventilation, aiding in the proper management of landfill fluids and gases or leakage detection. Additionally, these geocomposites serve as a protective layer, safeguarding geomembranes against puncture or damage.
• Roofing and deck pavement applications: Drainage geocomposites facilitate the efficient removal of water from surfaces, reducing the risk of water accumulation and moisturerelated damage.
• Drainage of sports fields: Drainage geocomposites effectively manage water. They help maintain optimal field conditions, prevent waterlogging and ensure consistent playability.
Subsurface drainage is the technique to control the flow of groundwater, through interception and/or deviation. An effective subsurface drainage system will reduce pore water pressures and increase effective stresses, thereby increasing the shear strength of the soil. Moreover, it will lessen the horizontal forces, traditional subsoil drain thus reducing overturning moments and the possibility of failure, as well as preventing the uncontrolled movement of soil particles (piping phenomena).
To ensure the successful implementation of subsurface drainage, groundwater must be removed in a controlled manner, without causing undue disturbance to the surrounding areas. This consideration becomes particularly important when the removal of groundwater may lead to movements or subsidence in the adjacent regions. Careful thought must be given to the positioning of the drainage system and the selection of appropriate materials to avoid any adverse consequences and maintain the stability of the surrounding areas.
Performance criteria
For a subsurface drain to be considered effective, it must meet the following three requirements:
1. The drainage system should be strategically placed and configured to intercept the seepage zone effectively. Proper consideration of the placement and layout of the subsurface drainage system is crucial to ensure it targets the intended area of seepage.
2. The subsurface drainage system should allow seepage water to enter with minimal resistance, while also minimising any disturbance or piping at the drain/soil interface. This is achieved by incorporating filters around the perimeter of the subsoil drains.
3. The subsurface drainage system must be designed to remove the required amount of groundwater from the soil in the required time interval. The dimensions of the drains, the selection of appropriate drainage media, and the configuration of the drainage system all contribute to its ability to perform satisfactorily.
Hydraulic gradient and pressure
The drainage capacity of a geocomposite depends on the hydraulic gradient as well as the pressure applied to, and the materials in contact with, the two faces. The design of a drainage system using geosynthetics is based on the evaluation of the drainage capacity available under actual operating conditions and the flow rate required by the project. The drainage capacity can be assessed based on appropriate laboratory tests, while the required flow rate is evaluated based on hydrological and hydraulic considerations. By considering these factors, an efficient and effective drainage system can be designed to meet the specific needs of the project.
Case study
In the case history presented below, the challenge arose when installing modular schools along 9th Road in Midrand, Gauteng. The site was characterised by saturated soft clayey soils with a water table higher than ground level, resulting in a natural spring.
The in-situ soil did not allow for pore water pressure dissipation in a short timeframe, posing difficulties for construction activities. The presence of excessive pore water pressure therefore made it unsuitable to install the modular school equipment on-site because the school would likely settle over time due to consolidation as the pore water pressure dissipated.
Traditional methods of draining the water and increasing the bearing capacity were not viable due to time restrictions. Importing soil to provide a stable foundation was also not feasible because the introduced soil would mix with the existing in-situ soil and fail under any load.
This situation presented a significant challenge for the contractor, who needed an alternative solution to install modular schools within time constraints. In response, this entailed the use of geosynthetic materials and techniques to address the challenges of saturated soft soil and a high water table.
in-situ soil, and provide drainage to dissipate excess water pressure, an 11 mm thick drainage geocomposite was utilised. This geocomposite consisted of a geotextile separation layer combined with a geomat core for effective drainage.
The approach involved placing the drainage geocomposite directly on top of the existing in-situ soil without earthworks. It was then covered with 300 mm imported material. Additionally, woven geosynthetic grids were incorporated into the solution to minimise longterm settlement (see Figure 3).
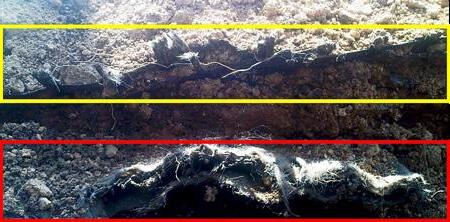
Within three days, 2 000 m2 of layer works were constructed using drainage geocomposites and geosynthetic grids. In contrast, a conventional solution would have required excavating and replacing at least 1.5 m of in-situ soil with imported material, along with the installation of a herringbone drainage system beneath the imported material. Such an approach would have increased costs and construction time.
Conclusion
Drainage geocomposites may be used in various applications to divert groundwater in a controlled fashion away from structures by filtering water through and transporting it away from the structures. Plus, the fact that drainage geocomposites can provide up to three times the drainage capacity with 60% less excavation when compared to conventional gravel drains is a clear advantage. This allows for an overall reduction in the construction cost and time in an environment where natural materials are inaccessible.
To avoid contamination of the imported material with the www.maccaferri.com/za
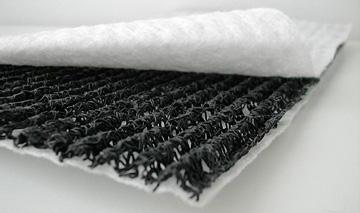