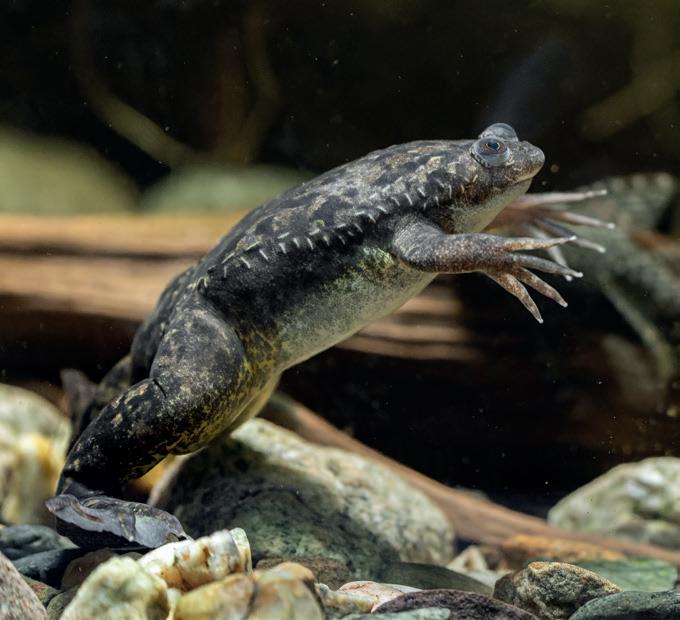
7 minute read
SHOCKING DISCOVERIES FOR LIMB RE-GROWTH
New scientific developments have found that human limb-regeneration treatments may be closer than we think.
Regeneration is a widely documented phenomenon, but one which is restricted to just a few species of animals. From deer that can re-grow antler skin, bones and nerves to axolotls that can re-grow organs and flatworms that can re-build their entire selves, the concept of ‘regeneration’ has been tantalising scientists for centuries. Not only do these species provide invaluable insight into biology itself, but the practical applications for a medicine designed to prompt limb regrowth are immense. Now, scientists are starting to discover that these obscure capabilities might not be limited to individual species. With the correct medication and a better understanding of bioelectricity, perhaps we could encourage limb re-growth in more species, including Homo sapiens.
Advertisement
The Levin Lab
Dr Michael Levin is a leading scientist in regeneration. As Co-Editor of the Bioelectricity Journal, Dr Levin and the Levin Lab at Tufts University are leading the charge when it comes to research in this field. Dr Levin told Exotics Keeper Magazine: “Bioelectricity is the phenomenon of cells communicating with each other electrically”. ‘Molecular Bioelectricity’ is a reasonably new field of research. Previously, ‘electrophysiology’ was the term used to describe the study of electric currents in tissues throughout the body. These were most closely associated with the nervous system and tracked the currents used to keep rhythms, patterns and behaviours generated by the brain and nervous system. Bioelectricity is something slightly different.
Dr Levin continued: “Usually people study this in neurons, but actually cells were doing this long before neurons evolved. In fact, evolution discovered the benefits of electric networks around the time of bacterial biofilms. Ions like potassium and sodium flow in and out of their surface, which gives them a voltage potential. Cells can propagate their voltage to each other, which allows the network to process information. While your brain uses bioelectricity to think about behaviour, your body tissues use it to make decisions about anatomical shape. Bioelectricity enables cells during embryogenesis to cooperate to build the right shape of the body with all the organs in the right place.”
Research at the Levin Lab is driven by a desire to understand the complexities that allow bodies to develop. “We all start life as an unfertilized egg - a bag of chemicals” added Dr Levin. “We eventually end up as sentient complex organisms with hopes and dreams, problem-solving, planning, and other cognitive capacities.
The process that slowly transforms a piece of physics and chemistry into an advanced mind is the most amazing aspect of all of science. And it uses the same mechanisms that brains use to implement minds. Amazing! This is what fascinates me, and the prospect of using this information to help people - fix birth defects, regenerate organs, reprogram cancer.”
Dr Michael Levin has been studying Developmental and Synthetic Biology since 1990 and is now a Distinguished Professor at Tufts University and Co-Director of the Institute for Computationally Designed Organisms. One of his pioneering breakthroughs in the field came in 2020 when he and his colleagues managed to re-grow the legs of Xenopus frogs, which are not usually capable of complex limb re-growth.
Limb re-growth
When some species of amphibian, particularly those that are aquatic, lose a limb they respond by growing a ‘blastema’. A blastema is group of undifferentiated cells at the edge of a wound that will give rise to new tissues during regeneration. Normally adult Xenopus form a bit of cartilage and skin at the end of the amputation wound. The scientists sought to affect the decision-making of those cells during the earliest part of the process and push them towards a leg regeneration cascade.
The materials required to kickstart this ground-breaking process are seemingly quite simple. Dr Levin continued: “There are 2 pieces. 1) A wearable bioreactor that goes onto the wound for 24 hours. This has a silk gel and keeps an aqueous, controlled environment around the wound. It keeps the payload, which is 2) the drugs needed to tell the wound cells what to do. In our case, they were 5 drugs that serve as a kickstart stimulus that tells the cells to ‘build whatever normally goes here.’ Our stimulus is there for 24 hours, while the cells are deciding what to do. After that, the leg grows for 18 months with no further input from us.”
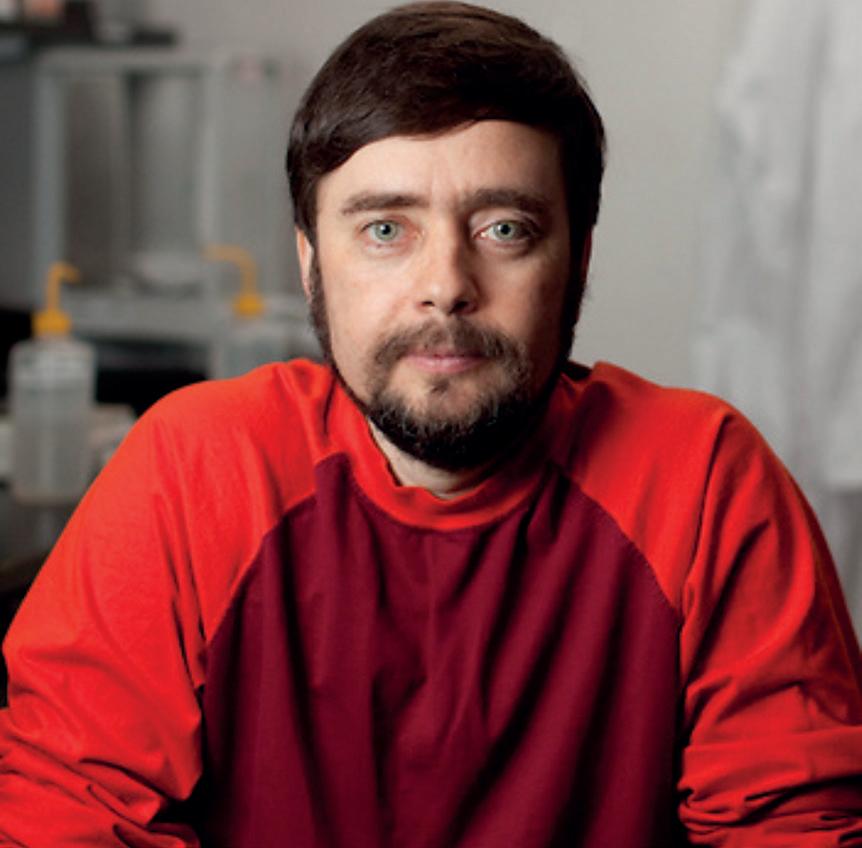
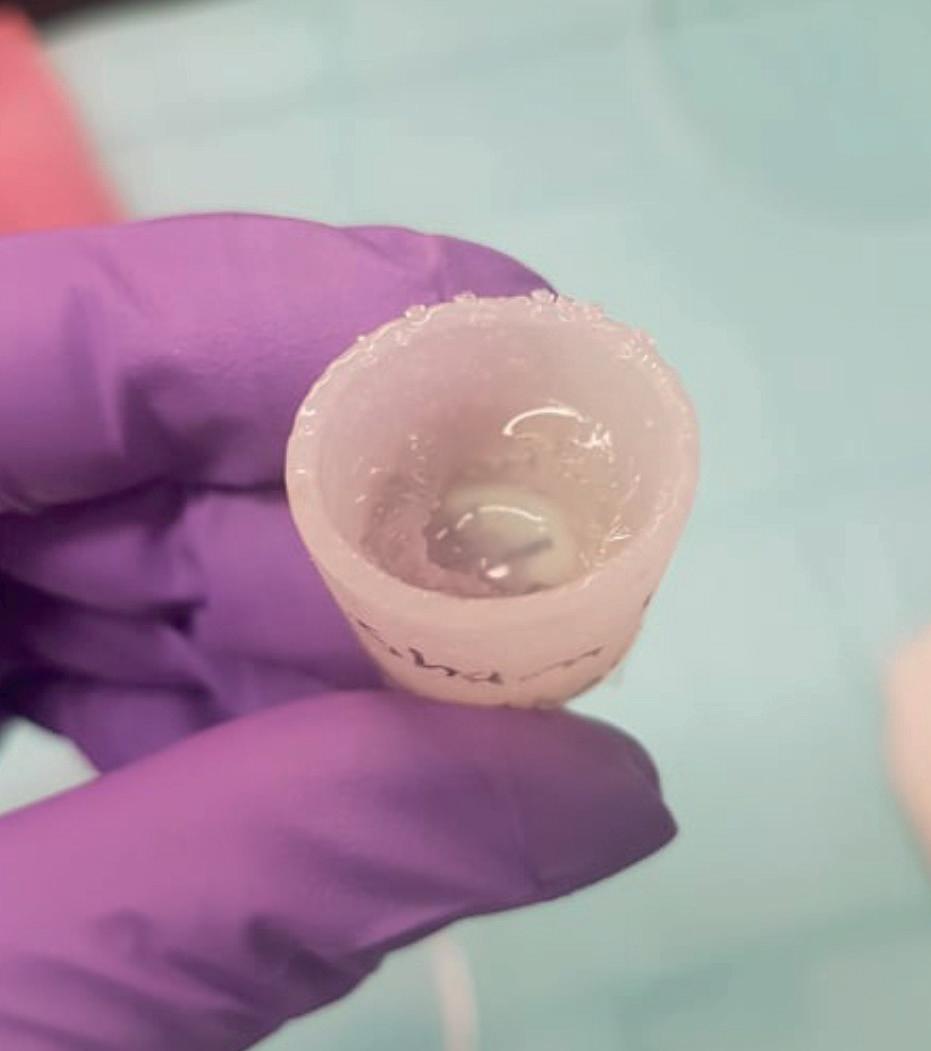
The cocktail of drugs was developed following previous success with a single drug, progesterone. This is a hormone released by the ovaries and is commonly used in combination with oestrogen to form the basis of contraceptive pills. It has also been used for various other fertility issues. This drug, when used with the bioreactor, prompted the frogs to grow a spiked limb which is still more developed than the blastema of other frogs. It was the addition of four extra drugs that kickstarted the regeneration of a fully functioning limb.
The ability to encourage a species to re-grow a limb holds huge potential for other taxa. The choice to use Xenopus frogs comes mostly out of practicality. “It's a very convenient model species” explained Dr Levin. “They live in our state-of-the-art facility and lay eggs frequently. The eggs develop into tadpoles right in front of your eyes, which offers scientists the ability to study every part of the growth and morphogenesis process. People use them to study neuroscience, immunology, cancer, kidney diseases, craniofacial syndromes, and much more.”
Whilst the lab is now researching how this technique can be applied to mammals, this unique insight into the world of reptiles and amphibians could have a whole host of veterinary and conservation-focused applications very soon.
Why amphibians?
Reptiles and amphibians are among the most famous classes of vertebrates to regenerate body parts. A whole plethora of lizard species can regrow their tails to varying degrees of complexity. Amphibians famously metamorphose from tadpoles into froglets in one of the most fascinating processes of life on Earth. American alligators (Alligator mississippiensis) can also grow up to 18% of their tail, including cartilage, blood vessels, nerves, and scales. Although these examples of re-growth are not considered fully-fledged ‘regeneration’, there are some species which are capable of growing complex limbs, complete with bone, tissues, and nerves such as axolotls (Ambystoma mexicanum).
Dr Levin continued: “Amphibians have better regenerative abilities than mammals, probably because evolution pushed mammals toward scaring. The reason is that unlike salamanders, our mammalian ancestors 1) lived in dry air (which makes it hard to drive bioelectric currents out of the wound epithelium), 2) walked on their limbs (which means they would put weight on the delicate regenerating cells and grind them into the forest floor while trying to regenerate), and 3) were prone to bleeding out and getting infected. One exception though is deer, which regenerate bone, nerve, vasculature, and velvet (skin) every year, at up to 1.5cm of new bone per day!”
These wonders of the natural world are continuously surprising scientists. As researchers continue to uncover how to trigger these dormant signals, it is highly possible that animals which have little or no regenerative capabilities may respond to the same five-drug cocktail and bioreactor combination used on the Xenopus frogs.
Retaining memories
Since these electric signals are inherent in many adult animals and hold the memory, or blueprint, of their design, more limb regeneration may be just around the corner. Dr Levin explained: “Bioelectricity is what holds the memories of what correct limbs and other organs are supposed to be shaped like and allows cell groups to form a collective intelligence that makes and repairs a complex body.” The memory-retention properties of bioelectricity can be seen clearly, not just in limb re-growth but in the cognitive abilities of some organisms.
One interesting example of regenerative ability is the planarian worm’s miraculous ability to retain memories even after it has been decapitated. This species of worm can be cut into hundreds of tiny pieces and will re-grow into hundreds of new planarian worms. The worms contain large populations of stem cells that have the potential to develop into whatever cell type the worm needs to regenerate, although much remains to be learned about how these anatomical building blocks become arranged in the right way to form a properly scaled and shaped planarian. In the 1800s it was thought that each cell could only inherit the genetic material to become a certain type of cell. In the 1890s Thomas Hunt Morgan dispelled this theory, as he cut a planarian worm into 279 pieces and created 279 new worms. This is still considered the record for the number of times the species can be cut up and regrown. How does a worm with no head eat? Well, the worm’s existing cells will self-destruct to give the animal enough nutrients to produce the more important cells, such as a head, and also to shrink the remaining piece down to match the small new head as it is produced. This newly grown head also contains original memories of the animal before it was severed.
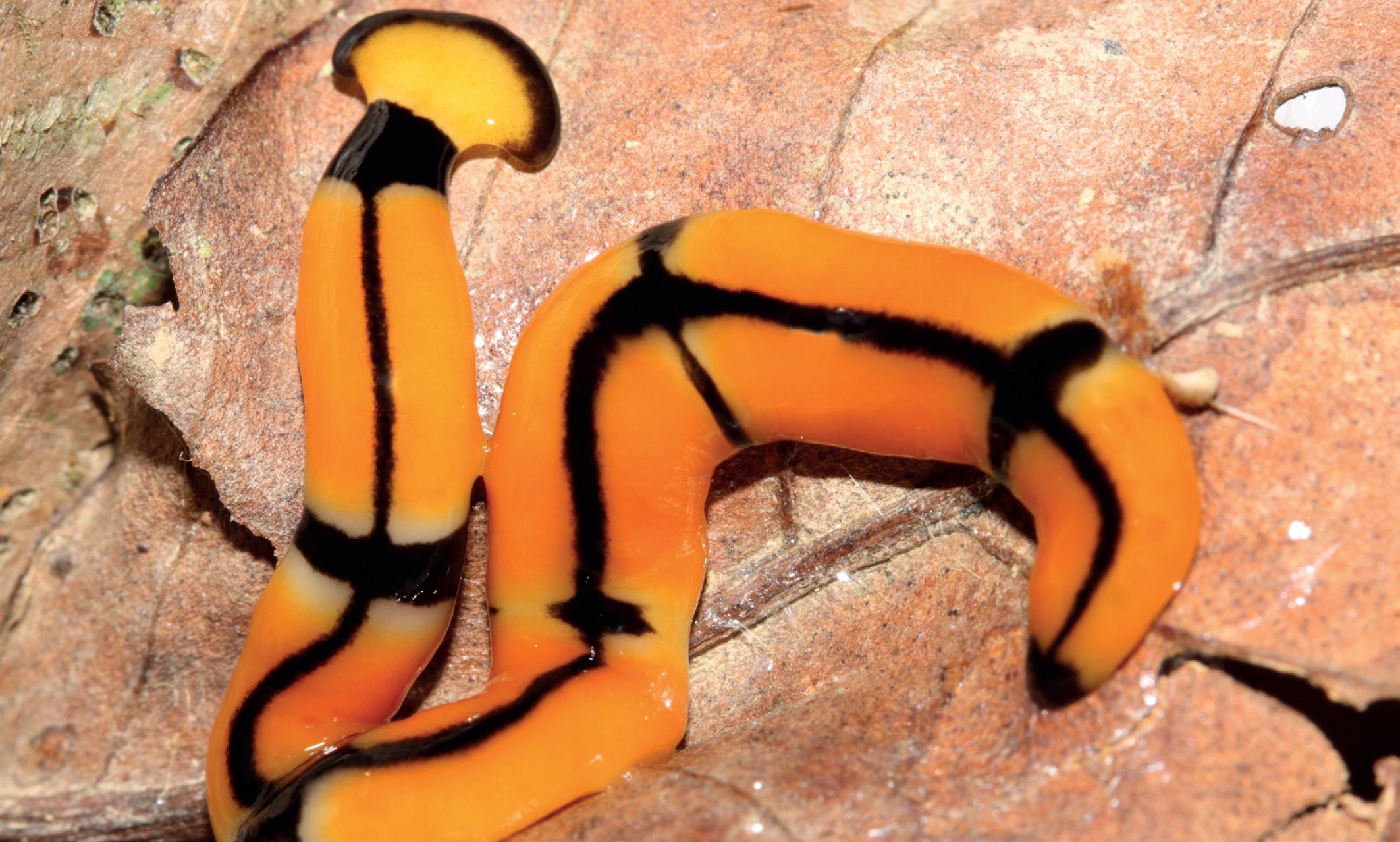
The future of bioelectricity
As people begin to understand bioelectricity better, the scope for potential medical applications is growing. Whilst regeneration in species such as axolotls has been widely documented, there are parallels which are now edging mankind ever closer to being able to grow new limbs. For example, human livers are capable of regenerating up to 50% of their original size, which is something of a phenomenon already. Clearly, our bodies already contain some level of bioelectric memory that allows us to re-grow certain parts.
Dr Levin added “We are all very closely related. The mechanisms we discover in Xenopus and other model species will help us understand how human bodies work as well. All of these things, including work in fruit flies, yeast, etc. lead to strategies for regenerative medicine. Someday, we will be repairing birth defects, regenerating damaged organs, normalizing tumours, and creating new synthetic living machines - all using information obtained by studying Xenopus and similar models.”
So, will we someday live in a world where human limb regrowth is commonplace? Dr Levin certainly thinks so. He concluded: “I don't know how long it will take, but I am convinced that someday we will be able to communicate with groups of cells, to tell them what they should build. At that point, we will be able to induce not only limb regrowth but any other organ we need.