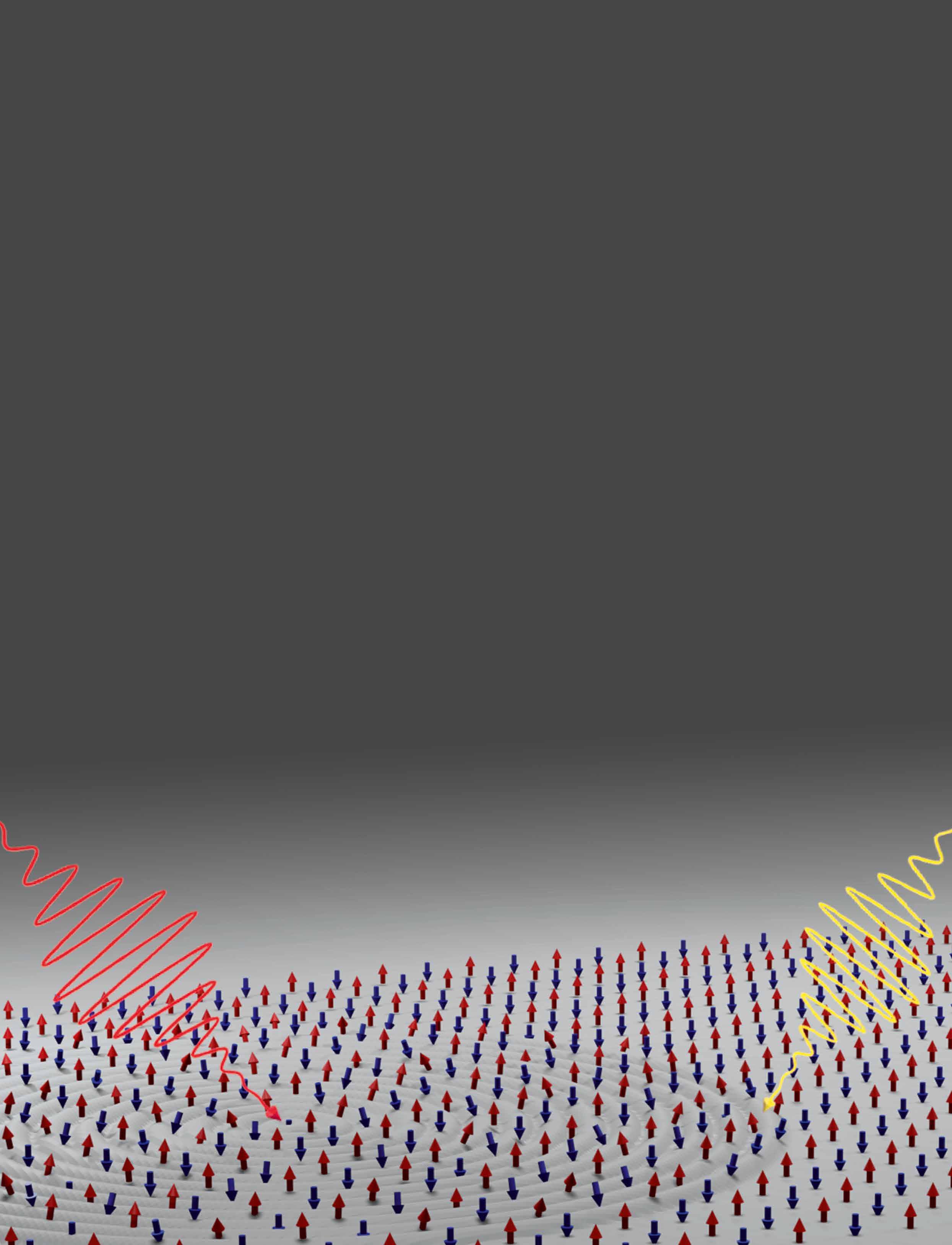
7 minute read
FALCON
Exciting a magnetic material with ultrashort laser pulses can strongly modify the macroscopic properties of the medium. Waves of the magnetic texture, called spin waves, can be induced in a broad frequency range, from a few GHz up to tens of THz. How do these spin waves behave? Can their propagation be controlled and manipulated on extreme time- and length-scales? These questions lie at the heart of Dr Davide Bossini’s research.
The behaviour of a magnetic solid can be described in great detail using the laws of thermodynamics as long as nothing changes, or if any changes occur very slowly. However, the so-called ultrafast phenomena, which occur on the picosecond (10-12 s) and femtosecond (10-15 s) timescales, cannot be described using thermodynamics. “This physical regime, in which the material is in nonequilibrium states, eludes thermodynamics, as this theory relies on the concept of equilibrium at its foundations,” says Dr Davide Bossini, a researcher at the University of Konstanz in Germany. This is a topic Dr Bossini is exploring within the framework of the FALCON project, in which he and his colleagues are investigating deeply fundamental questions around the properties of magnetic materials when perturbed on an ultrafast timescale. “We perturb solids using laser pulses with durations of tens of femtoseconds and measure how the system reacts in real time, aiming to manipulate its macroscopic properties in an ultrafast fashion,” he explains.
Ultrashort laser pulses and magnetic materials: A new frontier of science
This research involves changing the parameters of the laser pulses (wavelength, polarisation, intensity and duration) and investigating its effect on magnetic solids. While some of the phenomena that have previously been observed in this area of research could be grasped in a first approximation in terms of semithermodynamical pictures – others require the use of novel theoretical models. “These phenomena cannot be portrayed by established, well-defined canonical theories,” stresses Dr Bossini. “We have already observed that when we excite
a magnet under the proper conditions, collective coherent spin oscillations – even at frequencies exceeding 20 THz – can be triggered. We want to understand this phenomenon in depth, and to investigate if we can control the spatial propagation,” he explains. “Theories formulated to describe this peculiar regime of spin dynamics by us and other researchers suggest this phenomenon is intrinsically quantum mechanical. More precisely, quantum entanglement and squeezed states involving the photo-induced magnons have been theoretically proposed.”
There are not many cases of entanglement in solids so a direct experimental confirmation of the theoretical predictions would be a very interesting finding, a topic which Dr Bossini intends to investigate further outside the scope of his EmmyNoether project, in collaboration with his colleagues at the University of Konstanz. The theory can be paraphrased by stating that these two wave-like excitations are
entangled, so they can influence each other, despite not being physically connected. However, demonstrating the entanglement experimentally at the ultrafast timescale is a daunting challenge. “An experimental method to address this question in an unambiguous way is not available at the moment,” acknowledges Dr Bossini. “One of my activities in Konstanz, beyond FALCON, consists of an attempt at developing such a scheme, in tight collaboration with some colleagues at the Physics Department.”
macroscopic properties in an ultrafast fashion.
A highly sophisticated microscope is currently under development in the project, which is designed to detect the propagation of these spin waves. The aim is to achieve as high a spatial resolution as possible, in combination with the femtosecond temporal resolution provided by the duration of the laser pulses. “The challenge is to combine this time resolution, spatial resolution, and the ability to operate in cryogenic conditions in a single microscope,” explains Dr Bossini. The microscope will be used to study how both low-energy and high-energy spin waves propagate in space, while Dr Bossini also plans to investigate the possibility of coupling these waves to the charges of electrons. “If you want to exploit these ideas, to translate it into technological development, you must be able to couple any possible ultrafast magnetic manipulation to charges, as otherwise any possible novel concept could not be integrated with CMOS technology,” he says. “I mainly focus on coherent processes, as they they do not result in heating up electrons and the lattice of the material, while allowing us to store and manipulate information in the phase of the spin waves.”
Consequently metals are not suitable for this purpose. “As soon as you shine light on metals, you heat up the free electrons and, consequently, the lattice and the magnetic system. So we work with materials with an energy-gap, as insulators and semiconductors,” explains Dr Bossini. The focus for Dr Bossini and his group at the University of Konstanz is on fundamental research and he is in the ideal place to make interesting findings. “My host here in Konstanz (Prof. Alfred Leitenstorfer) specialises in building lasers with specifications which exceed commercial systems, in particular in the mid-infrared spectral range. I have the opportunity to work with these lasers,” he continues.
The complexity of the physics of ultrafast processes in magnetic media demands a tight collaboration between experimentalists and theorists. “I have recently begun collaborating with a group based here in Konstanz, and another in Mainz. We provide them with updates on our measurements,” outlines Dr Bossini. “We strive to understand how these spin waves propagate in space, and if we can couple them to charges. The goal is to couple not only low-energy but also high-energy spin waves with charges. We are going to investigate a variety of materials, which are promising for the identification of a coupling mechanism.”
This research also holds wider relevance to the development of a next-generation information technology, able to outperform the schemes employed nowadays, especially in big facilities. While solid state devices (SSDs) are commonly used in laptops, they are not used in the large-scale data centres run by major technology companies, as they are not available in sufficient volume and are not cost-effective. “Huge datacentres run by the likes of Google and Facebook rely on magnetic hard drives. A concept for a magnetic information technology, enabling not only the storage (write/read) but also the transfer of data on timescales thousands of times shorter than conventional hard disks – unconstrained by heating-related issues – is thus highly desirable,” says Dr Bossini. “This is the potential of investigating the optical generation of coherent magnons and the control of their spatio-temporal propagation on extreme scales.”
Femtosecond non-dissipAtive propagation controL and charge Conversion of THz antiferrOmagnetic magnoNs Project Objectives
Despite the feverishly growing research field investigating the interaction of light and quantum materials on extreme time- and length-scales, an optical drive and manipulation of the spatiotemporal dynamics on such scales of magnetic excitations has been so far not addressed. FALCON aims at setting a landmark in the realm of solid state physics, by establishing the all-optical generation, control and detection of extreme spatiotemporal dynamics of spin waves in magnets. During the process a novel experimental methodology and essentially novel physics will be developed, as the aimed extreme spatiotemporal regime is elusive of the state-ofthe-art condensed matter science. The results of FALCON will be key in assessing whether relying on collective magnetic excitations, i.e. spin waves, as information carriers can be the base of a novel paradigm of information technology.
Project Funding
The FALCON project is funded by the Deutsche Forschungsgemeinschaft (DFG).
Project Partners
• Prof. A. Leitenstorfer, University of
Konstanz, Germany • Prof. G. Cerullo, Politecnico di Milano, Italy
Contact Details
Dr. Davide Bossini Emmy Noether group leader Department of Physics University of Konstanz Universitätstraße 10 D-78457 Konstanz Box 696 Germany T: +49 (0)7531 88 4676 E: davide.bossini@uni-konstanz.de W: https://www.bossini.uni-konstanz.de/en/ team/dr-davide-bossini/start/
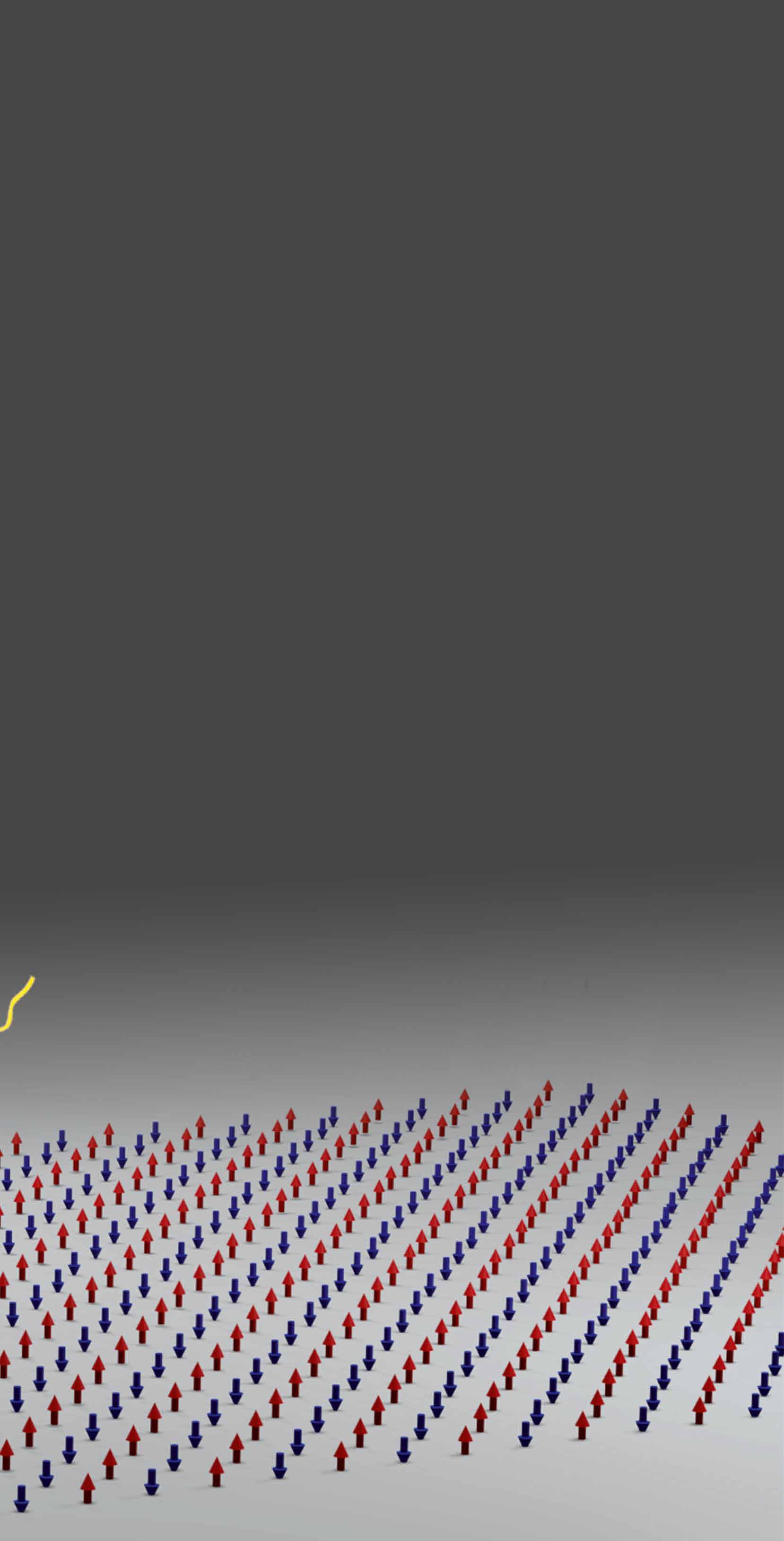
Dr Davide Bossini
Davide Bossini received his PHD in 2015 at Radboud University Nijmegen (NL). He spent 2 years at the University of Tokyo as a postdoc fellow. After 2 years as a Akademischer Rat auf Zeit, (equivalent to non-tenured assistant professor) at TU Dortmund, in 2020 he joined the University of Konstanz, as an Emmy Noether group leader.
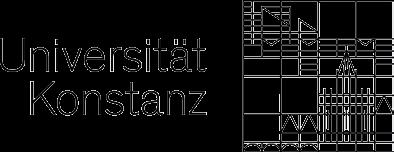
