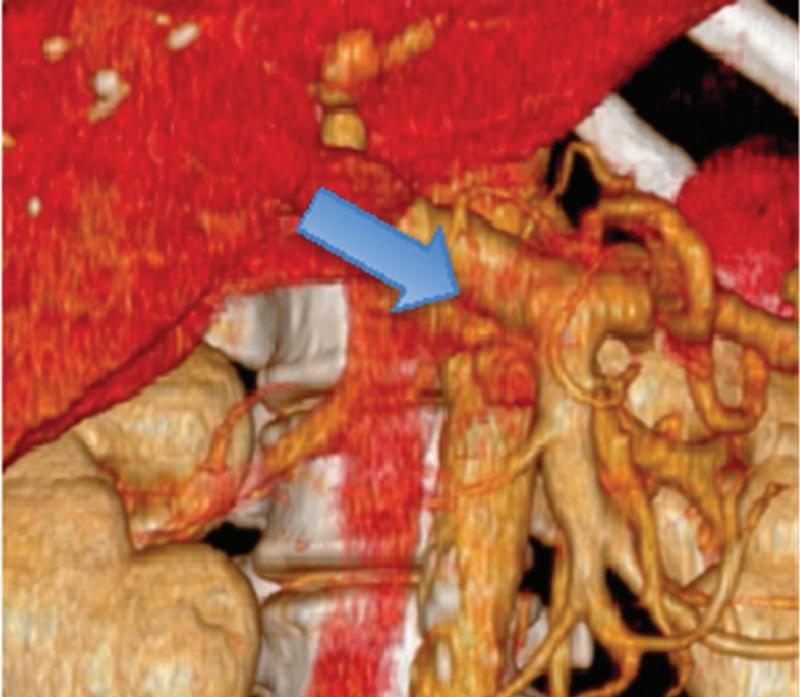
16 minute read
Spectral CT in oncology
Multi-detector computed tomography (MDCT) is an essential part of the armamentarium of oncologic imaging because of its availability, rapid acquisition time, and superior image quality. Dual-energy computed tomography (DECT) represents an innovative imaging technique, the basic principle of which lies in the analysis of the spectral dependencies of the net X-ray attenuation in two separate photon spectra during the CT acquisition, enabling the transition from density-based imaging to spectral imaging.
The IQon Spectral CT was installed in our radiology department in January 2017 and has been aiding our practice in patient pathological evaluations since. With the IQon Spectral CT, spectral information is obtained through a dual-layer spectral detector in addition to conventional CT density information. Using this technique, the low- and high-energy data sets are acquired simultaneously, without any time or spatial misregistration.1 Furthermore, the IQon Spectral CT captures this spectral information every time, without special planning or set-up. This means we can analyze the spectral data in any image retrospectively, which is very useful considering the high prevalence of unexpected incidental findings in clinical practice.2
The aim of this chapter is to provide an overview of the benefits and opportunities spectral CT provides in oncologic imaging, as well as to discuss the IQon Spectral CT utility for potential application in clinics based on our experience and reviewed literature.
Clinical applications
Spectral CT with a single acquisition allows the simultaneous generation of multiple data sets including:
• Different material-specific images: iodine mapping, virtual non-contrast (VNC) images, Z effective images, and calcium suppressed (CaSupp) images
• Energy-specific images: virtual monoenergetic (MonoE) images
The multi-parametric approach of spectral CT provides several advantages in oncologic imaging. The safety to patients for CT examinations is improved by reducing radiation dose and contrast medium administered, while at the same time, this approach provides advantages in tumor detection, characterization, and evaluation of response to therapy (Table 1).
Material-specific applications
Virtual non-contrast images
After material decomposition, the iodine content may be eliminated from the images, and VNC images can be generated. These images may replace the conventional unenhanced images, thereby decreasing the radiation burden to the patient significantly. This advantage is especially beneficial in young patients who are more at risk for radiation-induced consequences, or in patients who will undergo repeated follow-up CT examinations— a common scenario in oncologic imaging.
Data sets
Advantages of spectral CT
Replace unenhanced conventional acquisition
Radiation dose reduction*
VNC
Iodine mapping
Depict fat, cystic components/necrosis, blood, calcifications
Improve diagnostic confidence
Identify and quantify intralesional iodine uptake
May replace perfusion for evaluation of angiogenesis
Lesion characterization**
Lesion detection and characterization**
Predict and assess tumor response to therapy***
Radiation dose reduction*
Z effective and CaSupp
Monoenergetic
Improve diagnostic confidence
Improve lesion contrast and conspicuity (low Monoenergetic)
Low energy levels, closer to the k-edge of iodine, significant increase in mean attenuation of contrast-enhanced structures
Reduce metallic artifacts (high Monoenergetic)
Opportunity to analyze attenuation curves of different materials at distinct energy levels
*Safety: Radiation dose reduction; Amount of contrast material reduction
**Diagnosis: Lesion characterization; Lesion detection; Image quality
Lesion detection and characterization**
Lesion detection and characterization**
Amount of contrast material reduction*
Image quality**
Lesion characterization**
***Response to therapy assessment: Predict and assess tumor response to therapy
An increasing number of scientific papers in international literature has shown that VNC images represent a clinically feasible surrogate of conventional unenhanced images for various anatomic regions. This technique allows reliable assessment of pre-contrast lesion attenuation.3-9 Furthermore, VNC images are able to depict a broad range of different structural features that may be found in lesions, helping with lesion characterization including areas of low attenuation (e.g., fat, cystic components, or necrosis), intermediate attenuation (e.g., solid component or debris), and high attenuation (e.g., hemorrhagic or protein-rich content, calcifications).3,5-8,10,11
Despite the improvements seen in recent years in the image quality provided by DECT systems, there are still some limitations of VNC reconstructed images.1,12 First, VNC overestimates the HU of fat relative to conventional unenhanced images.4,5 In addition, in VNC, small calcifications may be suppressed since on VNC images a reduction in the size of calcifications has been found compared to the true unenhanced data sets.3,7 Radiologists should be aware of these limitations when incorporating VNC data sets into their clinical routine protocols.
Iodine mapping
Iodine images may be displayed as gray-scale images or as color coded overlay maps. Spectral CT can also provide a direct and accurate quantification of the iodine uptake in milligrams per milliliters (mg/ml). A lesion iodine concentration greater than 0.5 mg/ml has been suggested as highly indicative of lesion iodine uptake.8,13
Spectral CT-derived maps of iodine can be used to identify iodine uptake in tumors and can act as a surrogate for lesion vascularization. Beyond its diagnostic value for lesion detection and characterization (i.e., differential diagnosis of a lesion too small to be differentiated from a tiny cyst and/or a solid tumor/differentiation between a tiny cyst and a small solid tumor; evaluation of complex renal or adnexal cysts), iodine maps may also be beneficial for the assessment of lesion response after therapy. Iodine concentration quantification may also be useful for differentiation between benign and malignant lymph nodes, helping nodal staging.
Head and neck tumors. The use of iodine mapping in head and neck oncology imaging may improve lesion detection, increase lesion delineation,14 help to differentiate between benign and malignant tumors,15,16 or between tumor recurrence and post-therapeutic changes,17 as well as nodal staging.18,19 In a study by Kuno et al., iodine maps improved the accuracy of diagnosis of cartilage invasion in laryngeal and hypopharyngeal squamous cell carcinoma.14
The difficult task of detection for an underlying tumor as the cause of intracerebral hemorrhage is crucial. Preliminary results reported higher accuracy of DECT-derived color coded iodine images and virtual unenhanced images for detection of tumor enhancement compared to conventional post-contrast CT in this clinical scenario.20
Thoracic tumors. Iodine uptake has been used to characterize pulmonary nodules21 and to assess tumor grading and therapy response of thoracic tumors.22,23 DECT-derived maps of iodine distribution in the pulmonary parenchyma can also be used as a surrogate for pulmonary perfusion, which may be of particular interest in the preoperative evaluation of patients with lung cancer and in the assessment of pulmonary embolism as a common complication of malignancies.17,24
Liver tumors. Iodine maps improve visualization and detection of contrast uptake, potentially increasing the diagnostic confidence for assessment of metastatic liver lesion contrast enhancement or conversely ruling out the presence of any contrast enhancement in small benign cystic lesions.3,17 Atypical hemangiomas, which are frequently observed in patients with liver cirrhosis, represent an imaging challenge when attempting to differentiate them from hepatocellular carcinoma (HCC).17,25,26 Lv et al.25 demonstrated that lesion-specific iodine concentration could be used to differentiate hepatic hemangiomas from HCC. In addition, accurate detection and quantification of iodine concentration can be used as a more reliable and reproducible biomarker of tumor vascularity and may predict the likelihood of tumor response to antiangiogenic therapy.26-28 In a recent study, iodine density images from DECT provided good correlation with arterial perfusion images from perfusion CT in a group of HCC patients treated with sorafenib, with much lesser radiation dose (DLP 228 mGy*cm vs 1020 mGy*cm respectively).29 Iodine maps also have a diagnostic value in the assessment of HCC response to radiofrequency ablation30 or transarterial chemoembolization.31 Another potential advantage of iodine-quantification is the differentiation between malignant and benign thrombosis in patients with HCC and portal thrombus or vena cava invasion.32
Pancreatic tumors. Even when using an optimized protocol, approximately 11% of pancreatic ductal adenocarcinomas remain undetected on MDCT images because of the lack of a visible attenuation difference between the tumor and the adjacent pancreatic parenchyma.26
Because spectral CT-derived iodine images typically have higher CNRs, small or isoattenuating pancreatic ductal adenocarcinomas and cystic pancreatic neoplasms may be best seen on these images.17 In McNamara’s study of patients with small pancreatic ductal adenocarcinomas, subjective lesion conspicuity and readers’ confidence were highest with iodine images.33 Review of iodine images yielded additional information in 50% of cases in a population of 44 patients with focal pancreatic disease and proved useful for identifying the relationship of tumors with peripancreatic vessels.34 In Lin’s study of patients with small insulinomas, the use of iodine images together with low-energy images resulted in a further increase in detection from 87% with DECT low-energy image viewing alone and 87% with iodine image viewing alone, to 95.8%35 (Figure 1).
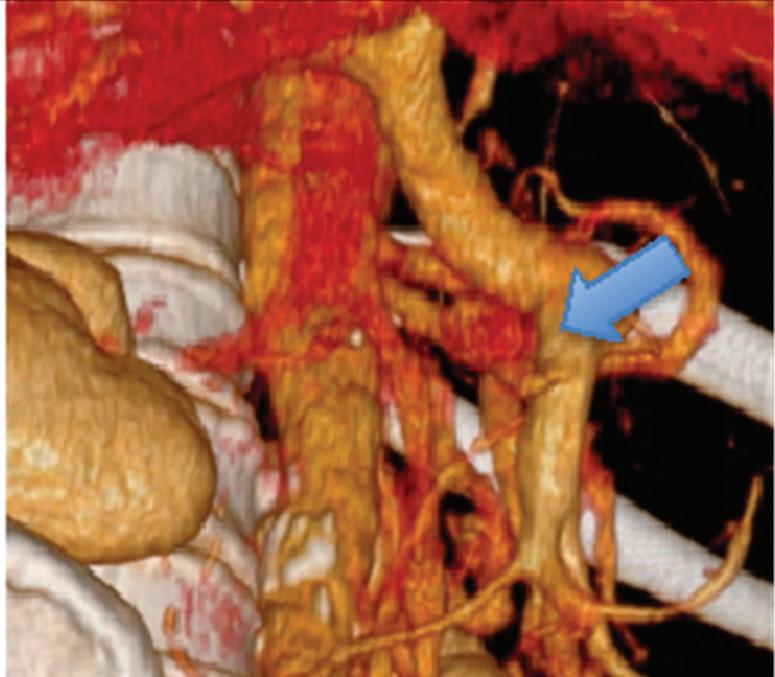
Gastrointestinal tumors. In gastric adenocarcinoma, quantitative iodine concentration (IC) measurements may be helpful to distinguish differentiated carcinoma from undifferentiated carcinoma, and metastatic lymph nodes from non-metastatic lymph nodes,36-37 and to assess response to neoadjuvant chemotherapy (NC). Tang et al. demonstrated that changes in the IC had more accuracy for predicting good response to NC than tumor thickness, with IC on the arterial phase being a better predictor than IC on the venous phase.38 Gastrointestinal stromal tumors (GIST), are extremely rare, corresponding to 1–3% of malignant gastrointestinal tumors, which are usually located in the stomach and small intestine. With the recent introduction of targeted therapy with imatinib, clinical management and prognosis of GIST patients have improved significantly.17 These new molecularly targeted drugs require different response criteria than the traditional tumor response criteria (e.g., WHO or RECIST), as several studies have suggested.38-40 Choi et al. have proposed the measurement of CT attenuation values as a potential indicator of GIST response in patients undergoing targeted therapy.41 Iodine-related attenuation was demonstrated to be a more robust response parameter than the Choi criteria, without the need of unenhanced acquisition.42 Iodine maps were demonstrated to be useful in detecting colorectal cancer without bowel preparation43 and in evaluating extracolonic spread of the tumor.44 In a recent study, enhanced DECT proved to be as accurate as MRI in differentiating between benign and malignant lymph nodes in rectal cancer.45
Neuroendocrine tumor of the head of the pancreas incidentally found during a CT examination with a single acquisition in the venous phase. (a) Coronal and (d) axial conventional images, (b, e) corresponding monoenergetic 50 keV images, and (c, f) Volume rendered (VR) reconstructions obtained from monoenergetic 50 keV images. Note the improvement in the conspicuity and delineation of the tumor (white arrow) in low monoenergetic images compared to the conventional ones. Multiplanar VR reconstructions rule out invasion of superior mesenteric-portal vein confluence (blue arrow).
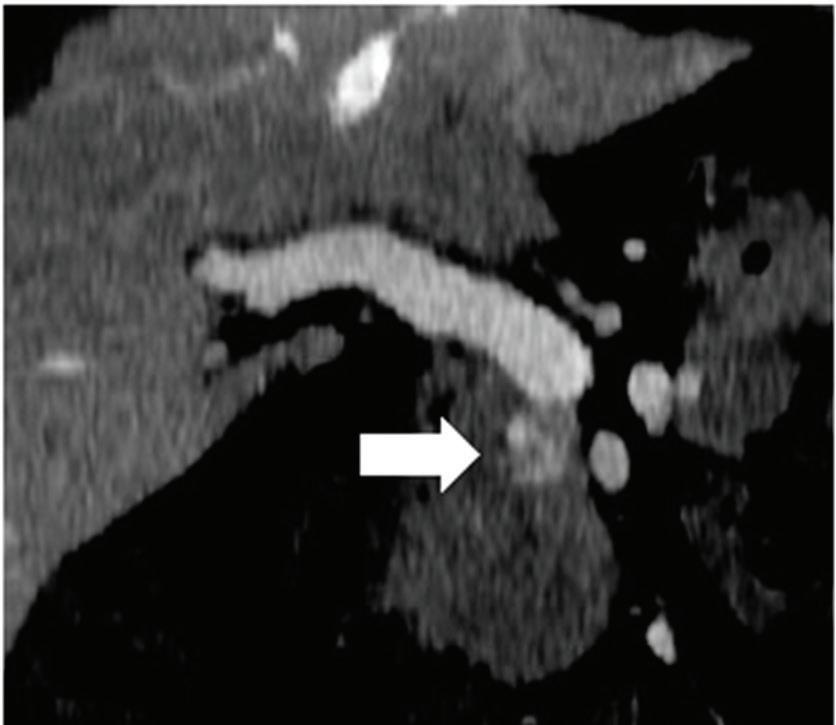
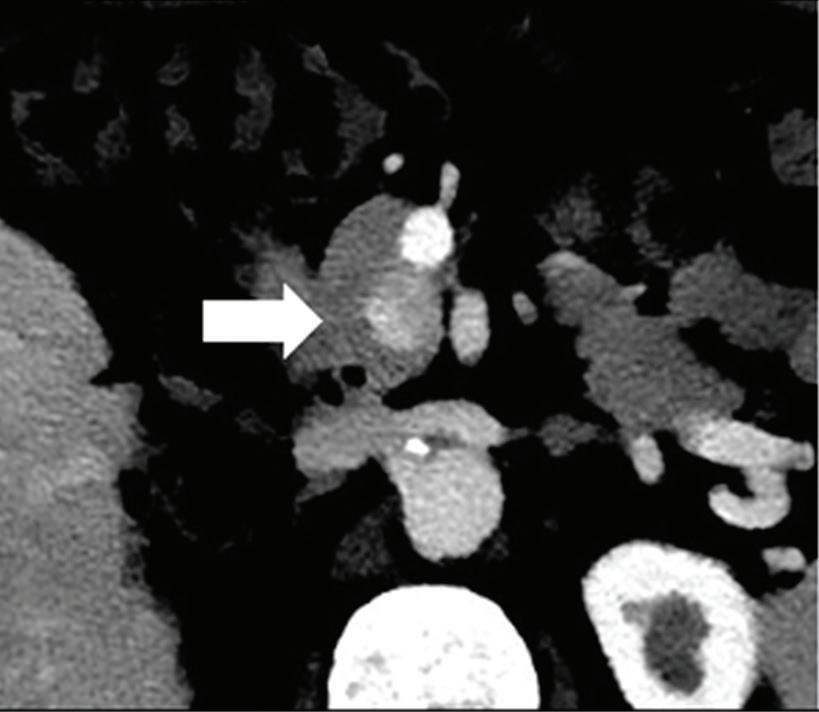
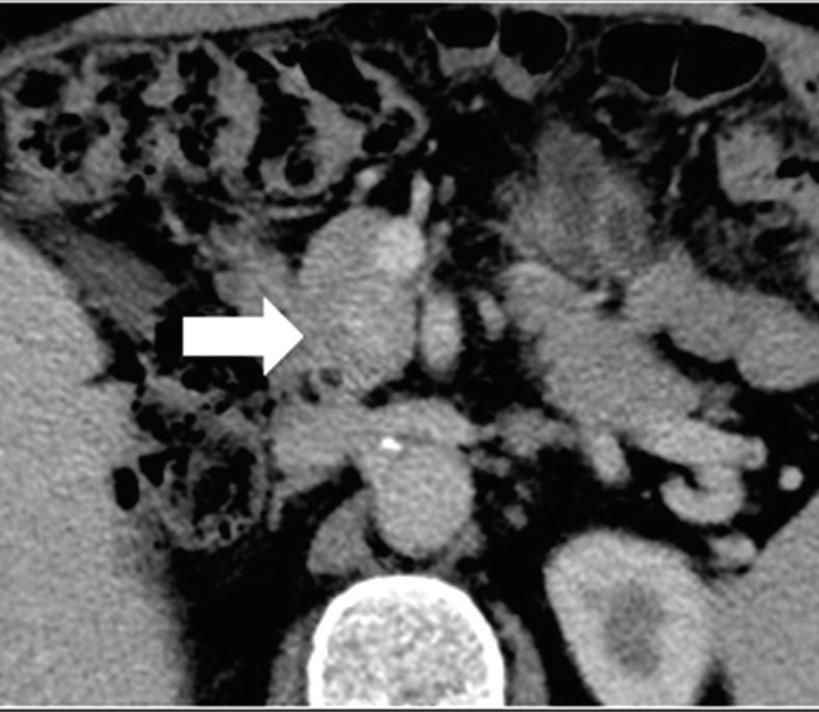
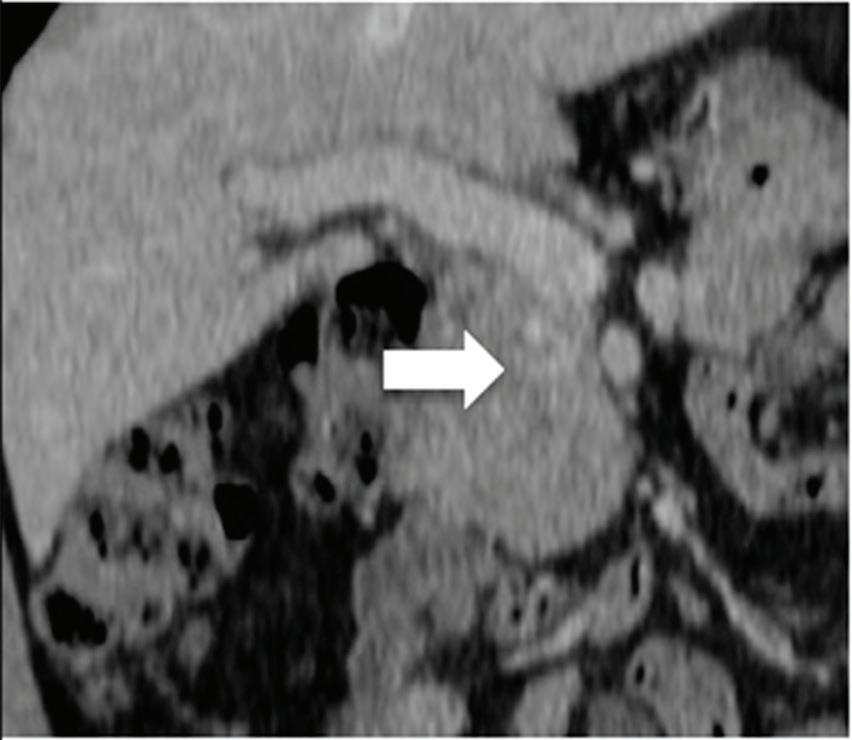
Renal and adrenal gland tumors. Contrast-enhanced-DECT images are particularly useful for the characterization of incidental renal or supra-renal lesions and for their follow-up, without the need of conventional unenhanced acquisition, translating into achieving a 30% mean dose savings for triphasic and up to 50% for biphasic renal protocols in daily clinical practice.17 It is also very useful in depicting hypervascular renal cell carcinoma metastasis in the liver or other sites, such as in the peritoneum and bowel wall (Figures 2 and 3).
Gynecologic tumors. Iodine images in gynecologic cancer are useful for characterization of a complex ovarian cyst (by detecting and quantifying subtle enhancing mural nodules or papillary projections) and for detection of enhancing subdiaphragmatic, intramuscular, infiltrating muscular, or bowel implants, which sometimes could not be differentiated from the surrounding tissues by the mean attenuation values.46 As previously stated, another important role of these data sets is the differentiation between benign and malignant lymph nodes.36,45,46
Z effective
Spectral CT is able to generate Z effective images that show the mean atomic number of the material present in a voxel (Z effective values) and can be displayed as color coded overlay maps. Z effective images may be helpful to enhance the visual differences between different tissue types.46 Furthermore, due to the high atomic number of iodine compared to other tissues present in the human body, the Z effective images can highlight enhancing structures (Figures 2, 3, 4, and 5) and could be used for quantitative analysis to differentiate benign from malignant tumors or lymph nodes.16,45,47
Calcium suppressed images
The IQon Spectral CT scanner is also able to generate virtual calcium suppressed (CaSupp) images. Kosmala et al. demonstrated an excellent diagnostic performance of visual and ROI-based analyses of dual-energy virtual non-calcium images for assessing bone marrow infiltration in patients with multiple myeloma (sensitivity of 93.3%, specificity of 92.4%, accuracy of 92.7%), with precision comparable to that of MR imaging.48
Energy-specific applications
Dedicated post-processing of DECT data sets also provide the possibility to reconstruct virtual monoenergetic (MonoE) images. The selected monoenergetic images should target the diagnostic task. Use of low monoenergetic images (40-60 keV), acquired closer to the k-edge of iodine, improves lesion detection and makes vascular studies possible with a significant reduction of the amount of contrast material administrated.49 On the other hand, high monoenergetic images (100-200 keV) provide less contrast but are useful to reduce artifacts caused by metal implants. This technique can also be used in the setting of tissue characterization by analyzing attenuation curves of different materials at distinct energy levels.17,50
Head and neck tumors. In a study on head and neck squamous cell carcinoma (SCC), reconstruction of monochromatic virtual images at 60 keV resulted in improvement of subjective image quality, higher tumor attenuation, and increased tumor contrast-tonoise ratio.51
Thoracic abnormalities. Delesalle et al. demonstrated that low virtual monochromatic images (≤ 60 keV) provide optimal diagnostic enhancement of the pulmonary arteries for diagnosing pulmonary embolism with only 25–35 ml of iodinated contrast material (iodine concentration 370 mg/ml).49 The role of spectral CT in chest tumors is discussed more in detail in the chapter dealing with thoracic disorders.
Effectiveness of spectral CT imaging for detection of hypervascular liver metastases. A small hypervascular metastasis (arrow) from renal cell carcinoma. (a) Axial contrast-enhanced conventional CT image obtained in the arterial phase, corresponding (b) monoenergetic 50 keV, and (c) Z effective image.
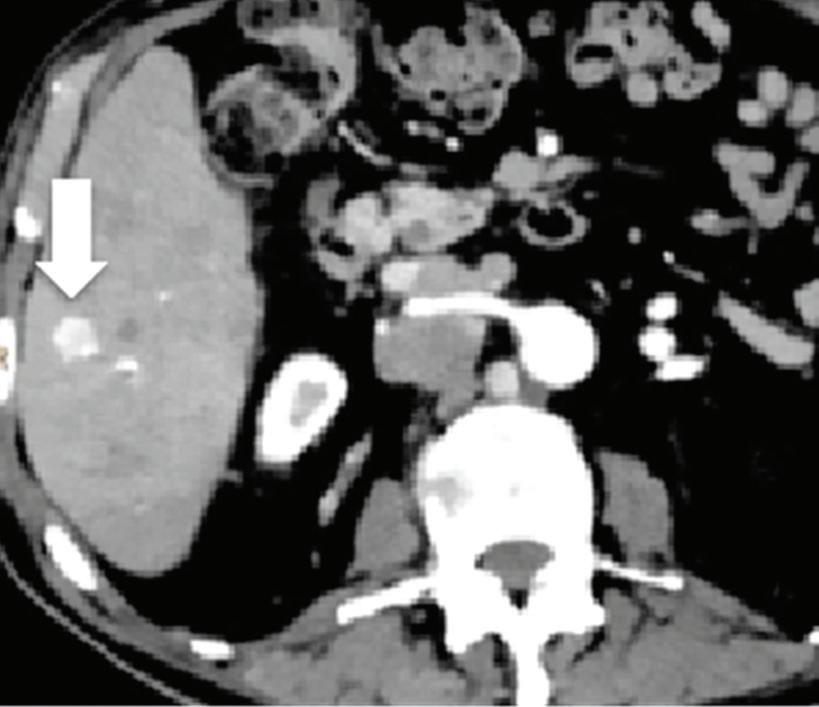
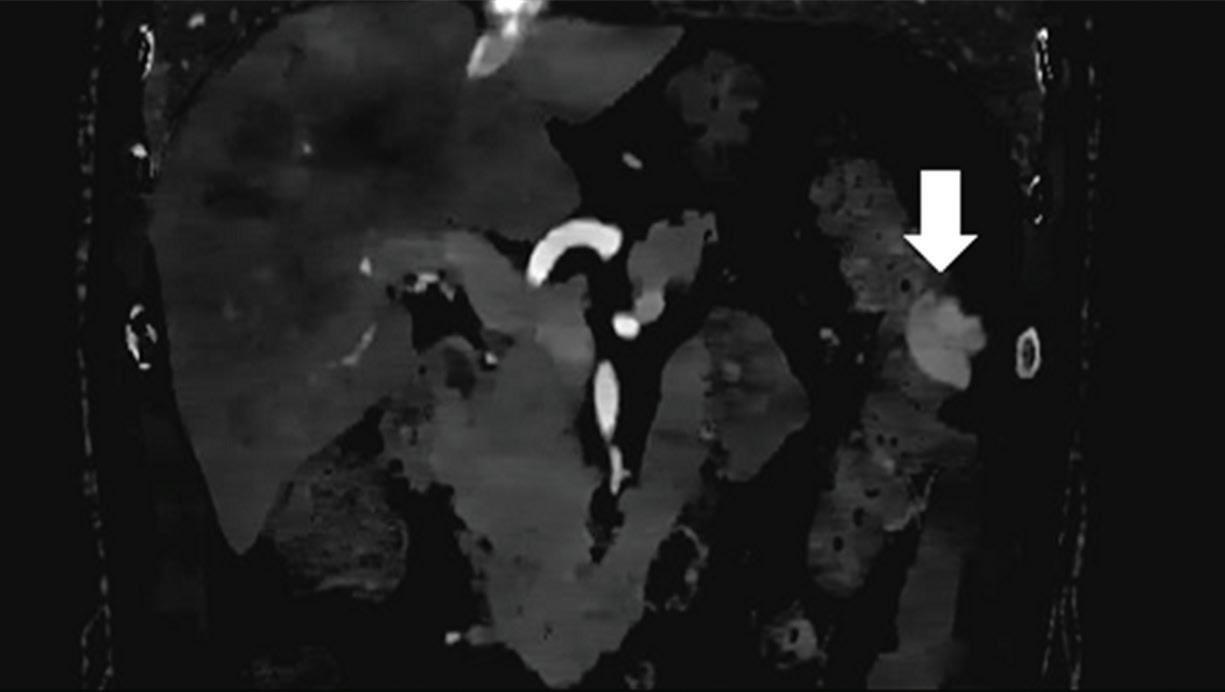
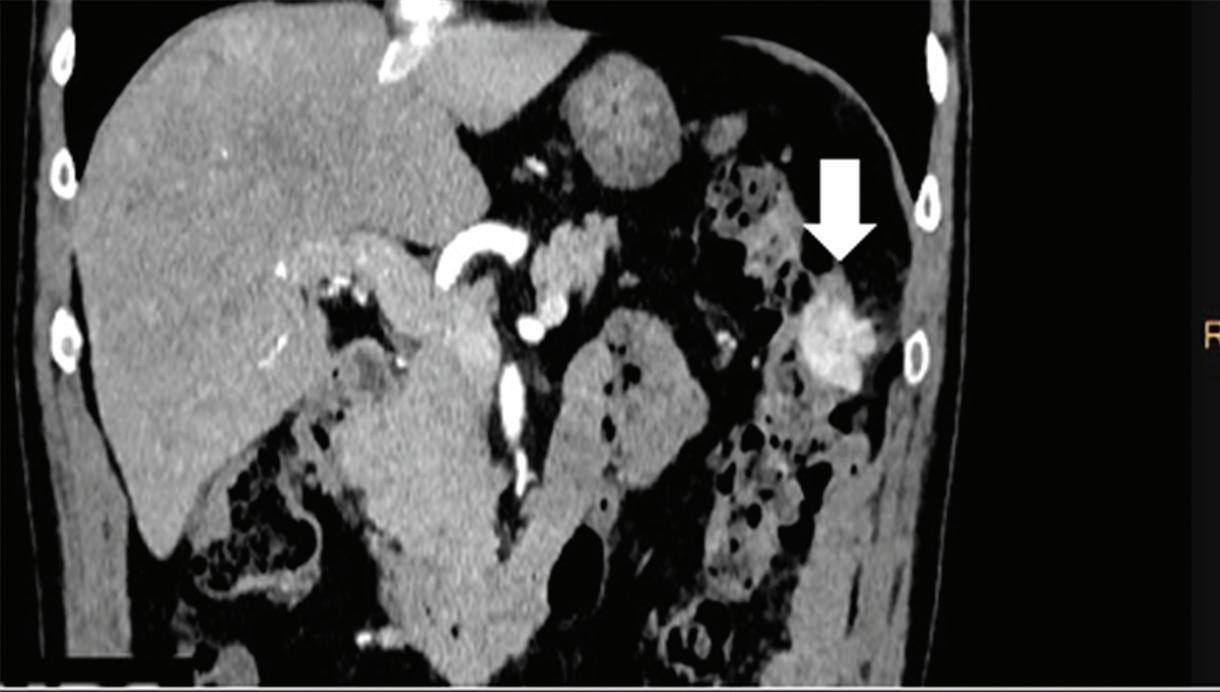
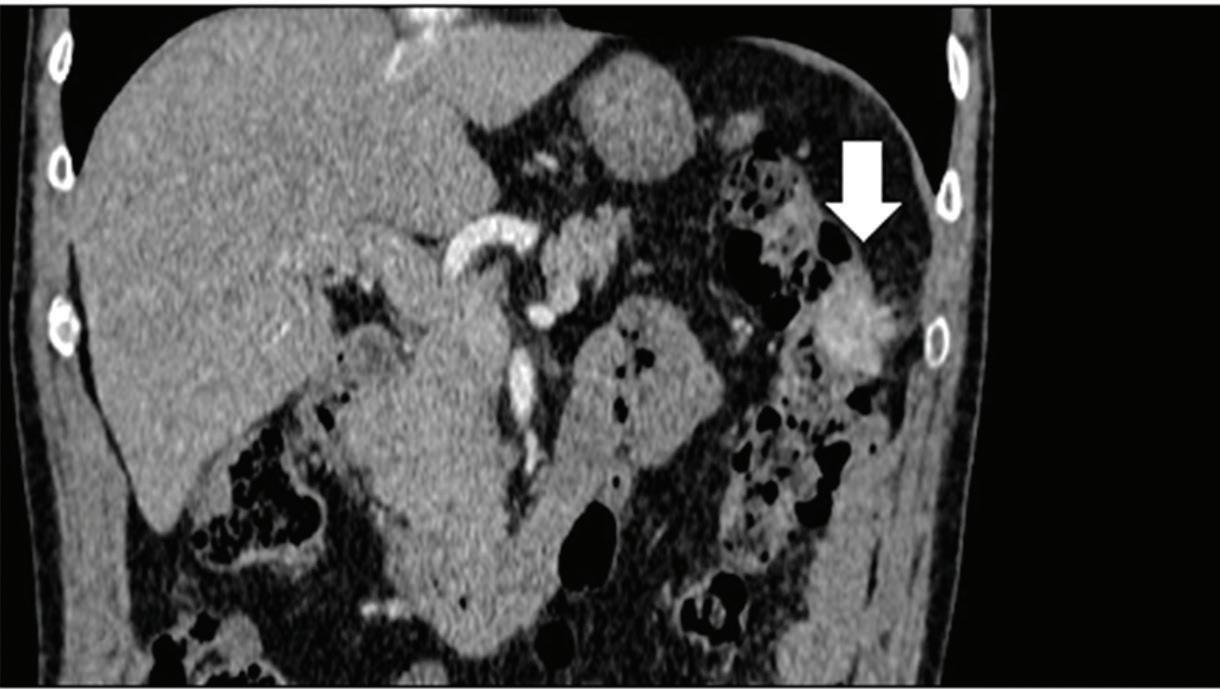
Effectiveness of spectral CT imaging for detection of a peritoneal implant from a renal cell carcinoma invading the bowel wall (arrow). (a) Coronal contrast-enhanced conventional CT image obtained in the arterial phase, corresponding (b) Z effective, (c) monoenergetic 50 keV, and (d) iodine-density image.
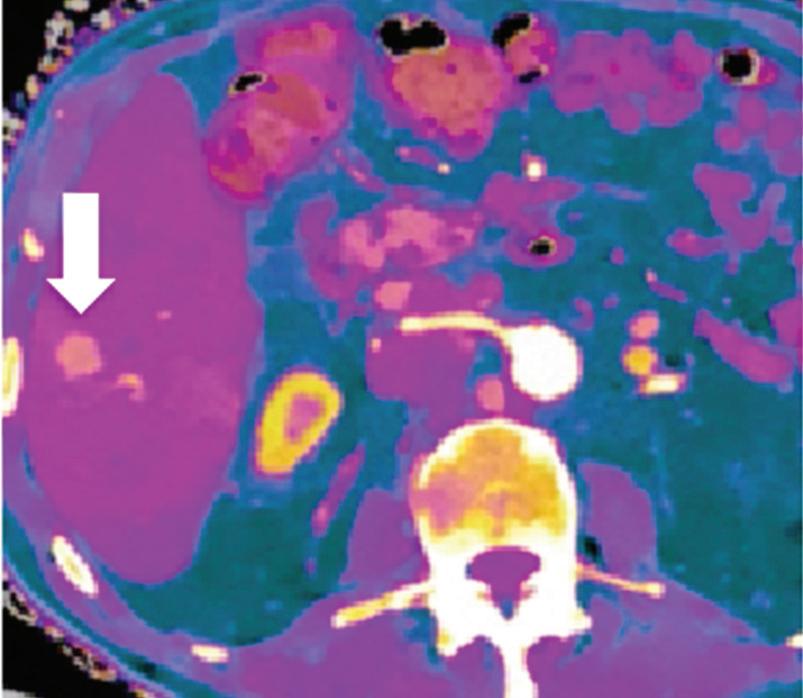
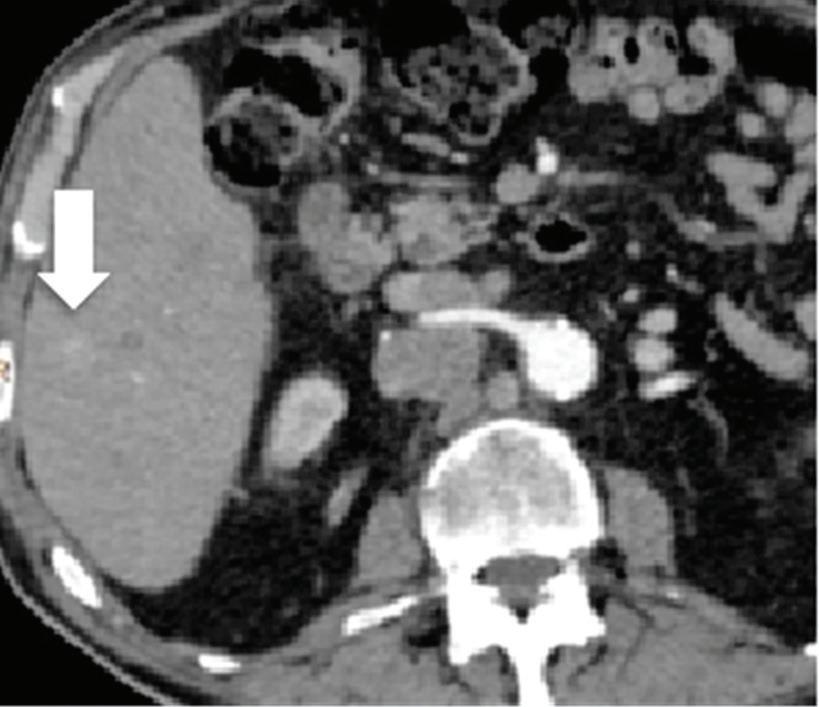
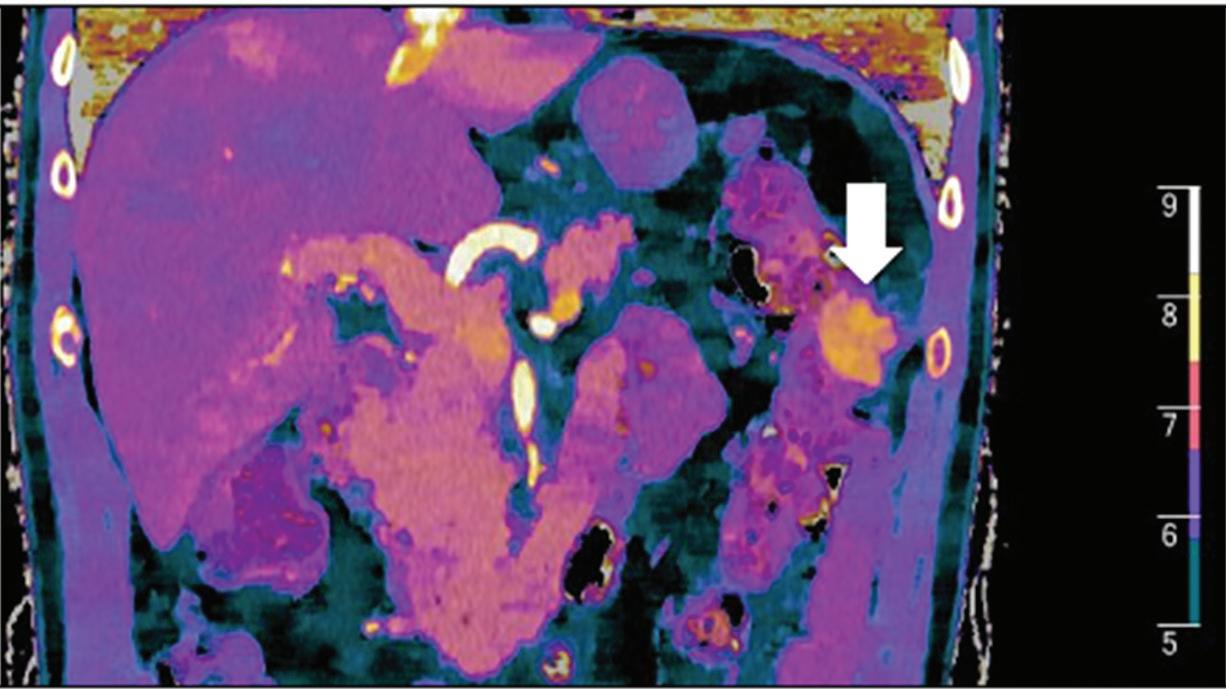
Multifocal HCC treated with sorafenib. (a) A small nodule (arrow) is barely seen on conventional axial CT image, acquired at late arterial phase after administration of 70 ml of contrast material (iodine concentration 350 mg/ml). (b) Monoenergetic 50 keV and (c) Z effective images improve the detection of this small nodule as well as allowing better evaluation of the peripheral solid component of both nodules seen in these images, with a precision comparable to that of (d) post contrast MR image.
A small isoattenuating pancreatic adenocarcinoma (arrow), (a) which tend to blend into normal parenchyma on conventional axial CT image, acquired at pancreatic parenchymal phase with administration of 70 ml of contrast material (iodine concentration 350 mg/ml). (b) Monoenergetic 50 keV and (c) Z effective images improve detection by increasing conspicuity and contrast between tumor and normal parenchyma.
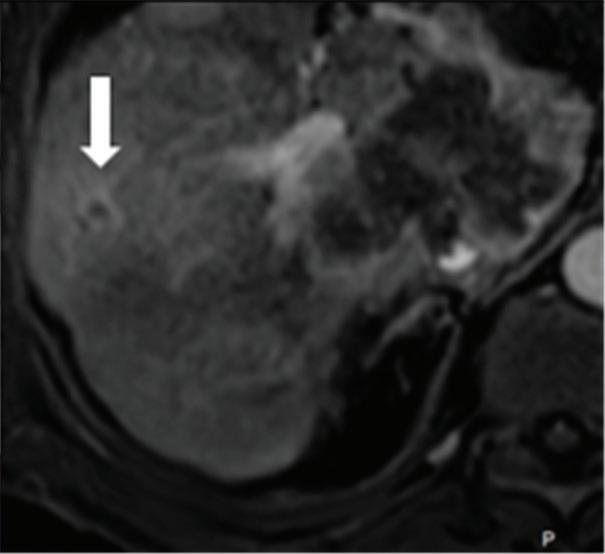
Liver tumors. Use of low-monoenergetic images on late arterial phase improves the detection of hypervascular liver lesions (e.g., HCC in cirrhotic liver) (Figure 4).3,17,52,53 It also improves detection of hypovascular liver metastases on portal-venous-phase.54
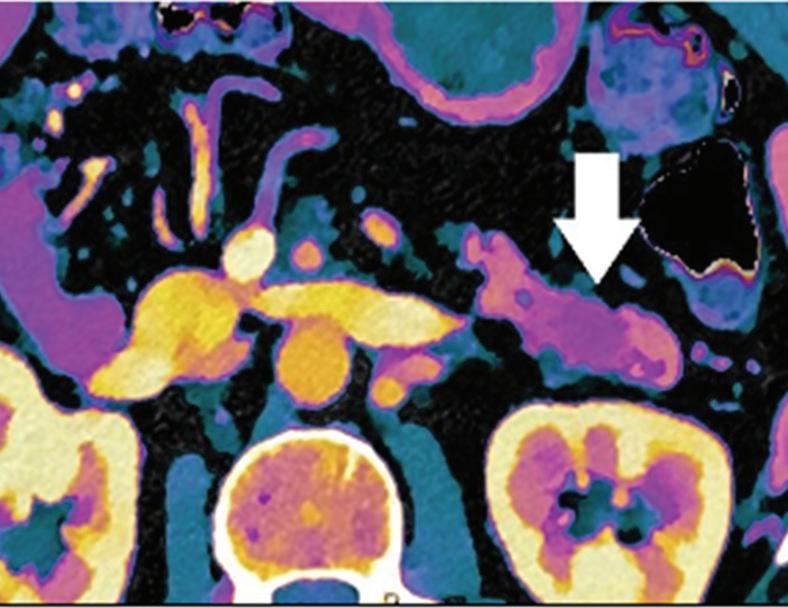
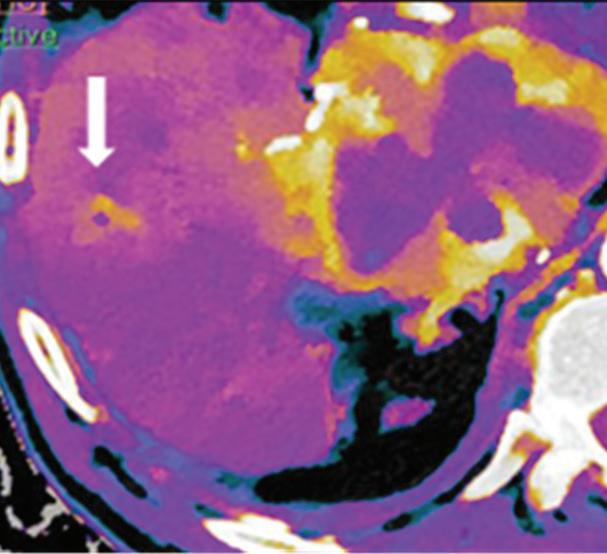
Pancreatic tumors. Several studies have demonstrated an obvious advantage of DECT for detection and staging of pancreatic adenocarcinoma using the low-monoenergetic data sets (Figure 5), regardless of the timing of the acquisition applied (single venous phase,55 pancreatic parenchymal phase,56 single split bolus single acquisition57).
Renal tumors. Low-monoenergetic images, as similar to iodine mapping, are useful for tumor detection, characterization, and assessment of tumor response to targeted therapy. Several studies demonstrated that renal lesions could be accurately characterized in a singlephase dual-energy protocol, with subsequent significant decrease in image interpretation time and radiation exposure.17
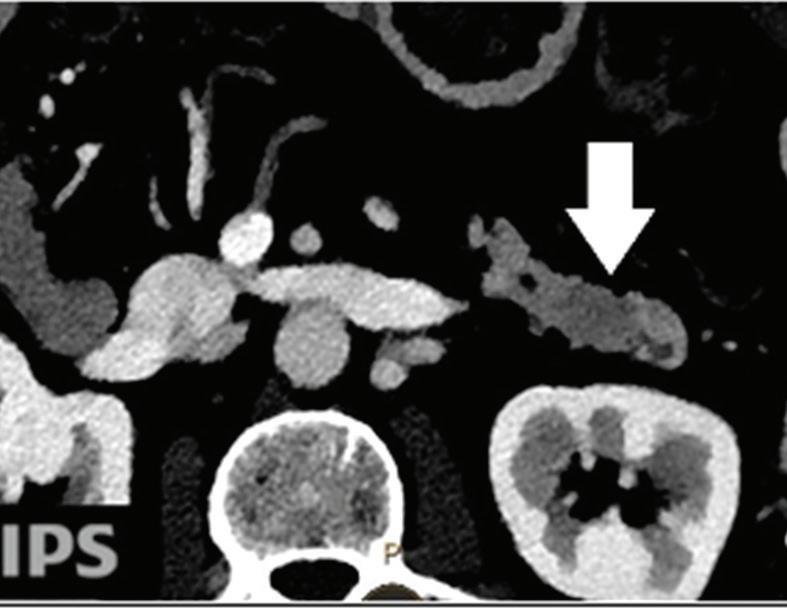
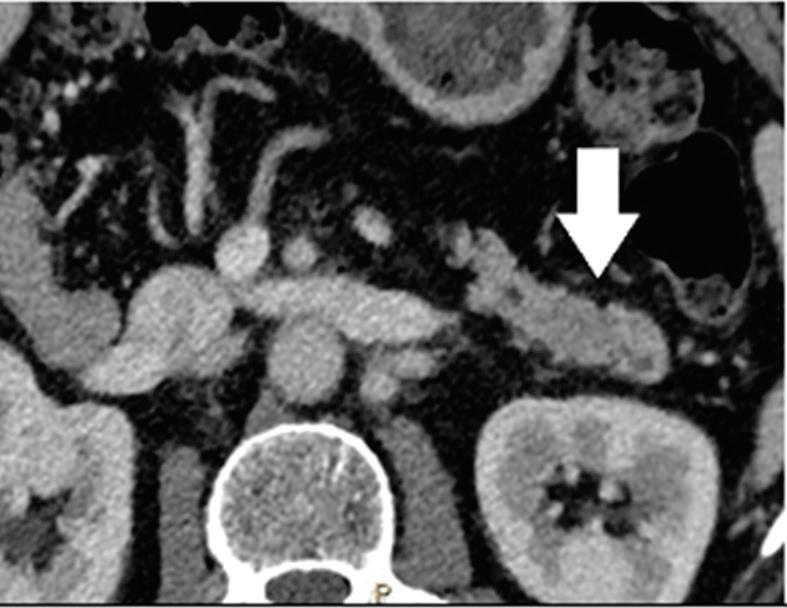
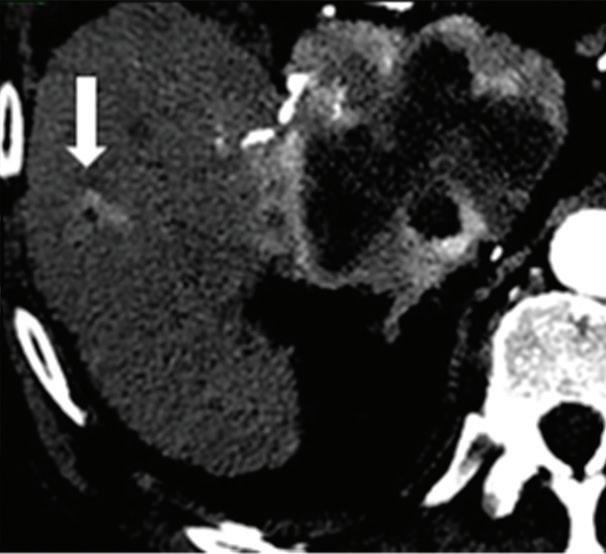
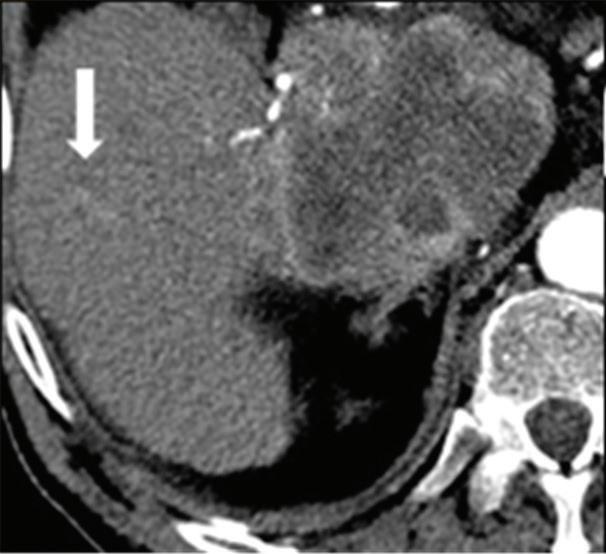
Conclusion
Spectral CT provides several beneficial applications in oncologic imaging. The possibility of obtaining different material-specific and energy-specific data sets during a single scan can improve detection and characterization of tumors. The safety of CT examinations may also be improved by reduction of radiation dose to the patient and the amount of administrated contrast material. In addition, this approach may be auxiliary in the prediction and evaluation of tumor response to therapy and in the detection of cancer-related comorbidities and complications.
References
1. McCollough CH, et al. Dual- and multi-energy CT: principles, technical approaches, and clinical applications. Radiology. 2015; 276: 637-653.
2. Gabbai M, et al. The clinical impact of retrospective analysis in spectral detector dual energy body CT. RSNA 2013, Chicago.
3. De Cecco CN, et al. Dual-Energy CT: Oncologic Applications. AJR. 2012; 199: 98-105.
4. Ananthakrishnan L, et al. Spectral detector CT-derived virtual non-contrast images: comparison of attenuation values with unenhanced CT. Abdom Radiol. 2017; 42: 702-709.
5. Ho LM, et al. Characterization of adrenal nodules with dual-energy CT: can virtual unenhanced attenuation values replace true unenhanced attenuation values? AJR. 2012; 198: 840–845.
6. De Cecco CN, et al. Dual energy CT (DECT) of the liver: conventional virtual unenhanced images. Eur Radiol. 2010; 20: 2870-2875.
7. Mileto A, et al. Pancreatic dual-source dual energy CT: is it time to discard unenhanced imaging? Clin Radiol. 2012; 67: 334-339.
8. Chandarana H, et al. Iodine quantification with dual energy CT: phantom study and preliminary experience with renal masses. AJR. 2011; 196: 693-700.
9. Pelgrim G J, et al. Accuracy of iodine quantification using dual energy CT in latest generation dual source and dual layer CT. Eur Radiol. 2017; 27: 3904-3912.
10. Ascenti G, et al. Dual-source dual energy CT evaluation of complex cystic renal masses. AJR. 2012; 199: 1026-1034.
11. Chae EJ, et al. Dual-energy computed tomography characterization of solitary pulmonary nodules. J Thorac Imaging. 2010; 25: 301-310.
12. De Cecco CN, et al. Virtual unenhanced images of the abdomen with 3rd generation dual-source dual-energy CT and 3rd generation iterative reconstruction: image quality, attenuation and radiation dose. RSNA 2014, Chicago.
13. Ascenti G, et al. Distinguishing enhancing from nonenhancing renal masses with dual-source dual energy CT: iodine quantification versus standard enhancement measurements. Eur Radiol. 2013; 23: 2288-2295.
14. Kuno H, et al. Evaluation of cartilage invasion by laryngeal and hypopharyngeal squamous cell carcinoma with dual-energy CT. Radiology. 2012; 265: 488-496.
15. Gao SY, et al. Identification of benign and malignant thyroid nodules by in vivo iodine concentration measurement using single-source dual energy CT. Medicine 2016; 95 (39): e4816.
16. Li M, et al. Dual-energy computed tomography imaging of thyroid nodule specimens: comparison with pathologic findings. Invest Radiol. 2012; 47: 58-64.
17. De Cecco CN, et al. Dual Energy in Oncology. Springer. 2015.
18. Tawfik AM, et al. Comparison of dual-energy CT-derived iodine content and iodine overlay of normal, inflammatory and metastatic squamous cell carcinoma cervical lymph nodes. Eur Radiol. 2014; 24: 574-580.
19. Liu X, et al. Papillary thyroid cancer: dual-energy spectral CT quantitative parameters of preoperative diagnosis of metastasis to the cervical lymph nodes. Radiology. 2014; 275: 167-176.
20. Kim SJ, et al. Dual-energy CT in the evaluation of intracerebral hemorrhage of unknown origin: differentiation between tumor bleeding and pure hemorrhage. AJNR. 2012; 33: 865-872.
21. Zhang Y, et al. Can spectral CT imaging improve the differentiation between malignant and benign solitary pulmonary nodules? PLOS ONE 2016|DOI: 10.1371/journal.pone.0147537.
22. Iwano S, et al. Evaluation of lung cancer by enhanced dual-energy CT: association between three-dimensional iodine concentration and tumour differentiation. Br J Radiol. 2015; 88 (1055).
23. Coche E. Evaluation of lung tumor response to therapy: current and emerging techniques. Diagnostic and Interventional Imaging 2016; 97: 1053-1065.
References
24. Otrakji A, et al. Dual-energy CT: spectrum of thoracic abdnormalities. Radiographics 2016; 36: 38-52.
25. Lv P, et al. Differentiation of small hepatic hemangioma from small hepatocellular carcinoma: recently introduced spectral CT method. Radiology. 2011; 259: 720-729.
26. Marin D, et al. State of the art: dual-energy CT of the abdomen. Radiology. 2014; 271: 327-342.
27. Chen B, et al. Precision of iodine quantification in hepatic CT: effects of iterative reconstruction with various imaging parameters. AJR. 2013; 200: 475-482.
28. Dai X, et al. Quantitative therapy response assessment by volumetric iodine-uptake measurement: initial experience in patients with advanced hepatocellular carcinoma treated with sorafenib. Eur J Radiol. 2013; 82: 327-334.
29. Gordic S, et al. Correlation between dual-energy and perfusion CT in patients with hepatocellular carcinoma. Radiology. 2016; 280: 78-87.
30. Lee SH, et al. Dual-energy computed tomography to assess tumor response to hepatic radiofrequency ablation: potential diagnostic values of virtual noncontrast images and iodine maps. Invest Radiol. 2011; 46:77-84.
31. Lee JA, et al. Dual-energy CT to detect recurrent HCC after TACE: initial experience of color-coded iodine CT imaging. Eur J Radiol. 2013; 82: 569-576.
32. Qian LJ, et al. Differentiation of neoplastic from bland macroscopic portal vein thrombi using dual-energy spectral CT imaging: a pilot study. Eur Radiol. 2012; 22: 2178-2185.
33. McNamara MM, F et al. Multireader evaluation of lesion conspicuity in small pancreatic adenocarcinomas: complimentary value of iodine material density and low keV simulated monoenergetic images using multiphasic rapid kVp-switching dual energy CT. Abdom Imaging. 2015; 40:1230-1240.
34. Chu AJ, et al. Dual-source, dual-energy multidetector CT for the evaluation of pancreatic tumours. Br J Radiol. 2012; 85: 891–898.
35. Lin XZ, Wu ZY, Tao R. Dual energy spectral CT imaging of insulinoma-value in preoperative diagnosis compared with conventional multi-detector CT. Eur J Radiol. 2012; 81:2487–2494.
36. Pan Z et al. Gastric Cancer Staging with Dual Energy Spectral CT Imaging. PLOS ONE 2013; 8 (2): e53651.
37. Liang P, et al. Iodine concentration in spectral CT: assessment of prognostic determinants in patients with gastric adenocarcinoma. AJR. 2017; 209: 1033-1038.
38. Tang L, et al. Evaluating the response of gastric carcinomas to neoadjuvant chemotherapy using iodine concentration on spectral CT: a comparison with pathological regression. Clinical Radiology. 2015; 70: 1198-1204
39. Benjamin RS, et al. We should desist using RECIST, at least in GIST. J Clin Oncol. 2007; 25:1760-1764
40. Meyer M, et al. CT-based response assessment of advanced gastrointestinal stromal tumor: dual energy CT provides a more predictive imaging biomarker of clinical benefit than RECIST or Choi criteria. Eur J Radiol. 2013; 82: 923-928.
41. Choi H, et al. Correlation of computed tomography and positron emission tomography in patients with metastatic gastrointestinal stromal tumor treated at a single institution with imatinib mesylate: proposal of new computed tomography response criteria. J Clin Oncol. 2007; 25: 1753-1759.
42. Apfaltrer P, et al. Contrast-enhanced dual-energy CT of Gastrointestinal Stromal Tumors: is iodine-related attenuation a potential indicator of tumor response? Invest Radiol. 2012; 47: 65-70.
43. Boellaard TN, et al. The feasibility of colorectal cancer detection using dual-energy computed tomography with iodine mapping. Clin Radiol. 2013; 68:799-806.
44. Chen CY, et al. Utility of the iodine overlay technique and virtual non-enhanced images for the preoperative T staging of colorectal cancer by dual-energy CT with tin filter technology. PLOS ONE 2014; 9, e113589.
45. Al-Najami I, et al. Dual-energy CT can detect malignant lymph nodes in rectal cancer. Eur Radiol. 2017; 90: 81-88.
46. Benveniste AP, et al. Potential application of dual-energy CT in gynecologic cancer: initial experience. AJR. 2017; 208: 695-705.
47. Papadakis A, Damilakis J. Spectral CT: On the accuracy of concentration and effective atomic number estimation. Phisic Medica. 2016; 32: 232-233.
48. Kosmala A, et al. Multiple myeloma and dual-energy CT: diagnostic accuracy of virtual noncalcium technique for detection of bone marrow infiltration of the spine and pelvis. Radiology. 2018; 286: 205-213.
49. Delesalle MA, et al. Spectral optimization of chest CT angiography with reduced iodine load: experience in 80 patients evaluated with dual-source, dual-energy CT. Radiology. 2013; 267: 256-266.
50. Agrawal MD, et al. Oncologic applications of dual-energy CT in abdomen. Radiographics. 2014; 34: 589-612.
51. Wichmann JL, et al. Virtual monoenergetic dual-energy computed tomography: optimization of kiloelectron volt settings in head and neck cancer. Invest Radiol. 2014; 49: 735-741.
52. Marin D, et al. Hypervascular liver tumors: low tube voltage, high tube current multidetector CT during late hepatic arterial phase for detection – initial clinical experience. Radiology. 2009; 251: 771-779.
53. Altenbernd J, et al. Dual- energy-CT of hypervascular liver lesions in patients with HCC: investigation of image quality and sensitivity. Eur Radiol. 2011; 21:738-743.
54. Yamada Y, et al. Virtual monochromatic spectral imaging for the evaluation of hypovascular hepatic metastases: the optimal monochromatic level with fast kilovoltage switching dual-energy computed tomography. Invest Radiol. 2012; 47: 292-298.
55. Macari M, et al. Dual-source dual-energy MDCT of pancreatic adenocarcinoma: initial observations with data generated at 80 kVp and simulated weighted-average 120 kVp. AJR. 2010; 194: 27-32.
56. Patel BN, et al. Single-source dual-energy spectral multidetector CT of pancreatic adenocarcinoma: optimization of energy level viewing significantly increases lesion contrast. Clin Radiol. 2013; 68:1 48-154.
57. Brook OR, et al. Split-bolus spectral multidetector CT of the pancreas: assessment of radiation dose and tumor conspicuity. Radiology. 2013: 269:139–148.
History Benefits or pitfalls of dual-energy CT