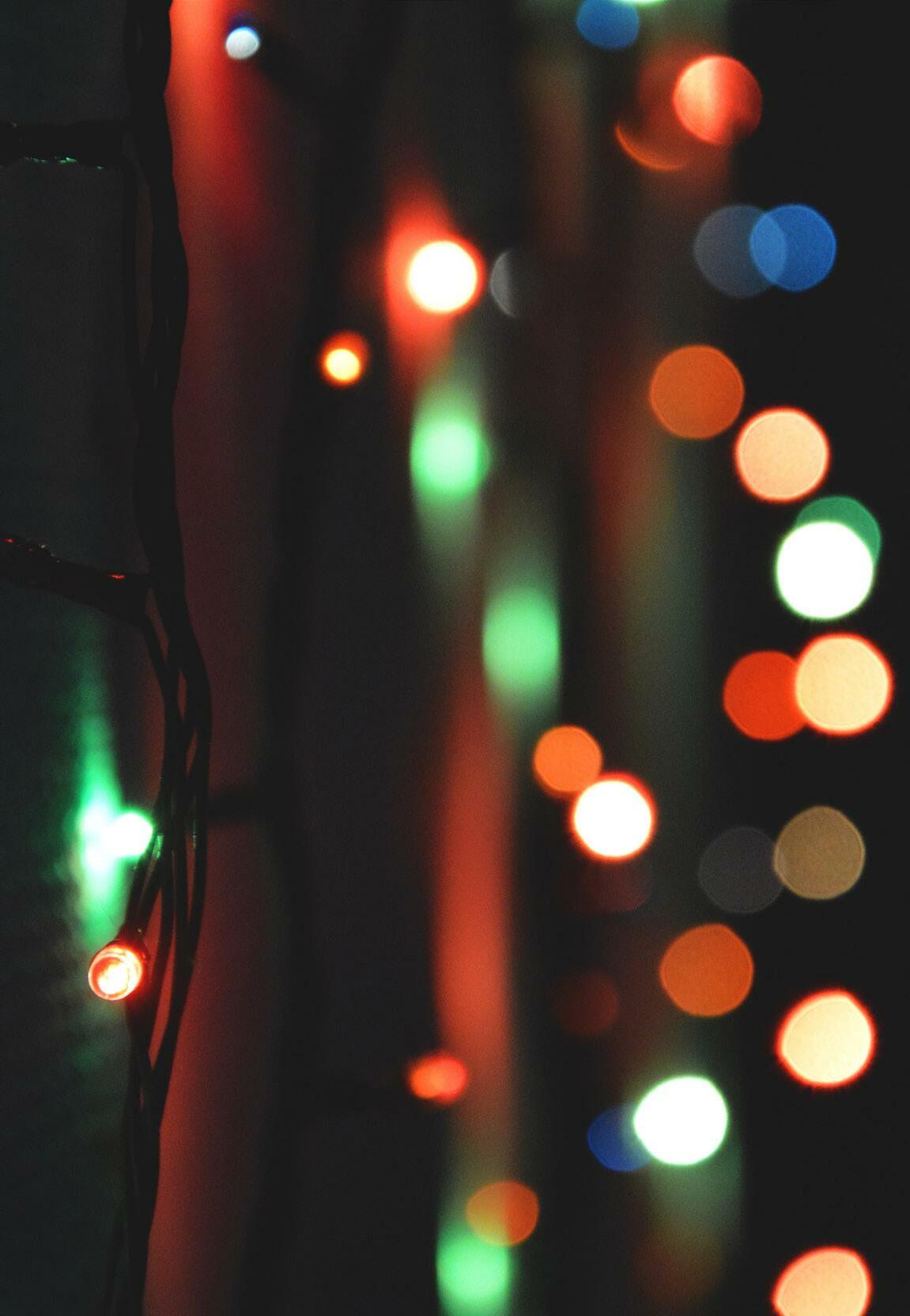
6 minute read
THE LANTHANIDES Dr Louise Natrajan
THE LANTHANIDES
Dr Louise Natrajan
‘Shining Light’ on the Chemistry of F-element Compounds
Often displayed at the bottom of the Period Table of the Elements, lies an intriguing group of elements, the lanthanides, or the 4f-elements (Figure 1). These are largely ignored until more advanced levels at degree level Chemistry as they exist due to filling up of electrons in the f-orbitals (which come after the d-orbitals in the transition metal groups). Historically, these elements were discovered much later on than the main parts of the Periodic Table and although they possess no known biological function in the body unlike most of the transition metals (e.g. iron in blood and vanadium as a trace element for life), they are indispensable commodities for modern life and technology as we know it today. The lanthanide elements are often called the ‘rare earth elements’ due to the fact their natural abundance in the Earth’s crust is concentrated in geographical areas including Scandinavia, China and Australia. However, their overall actual natural abundance is similar to that of bromine, tungsten, tin and arsenic. The only exception to this is the element promethium (Pm), which is radioactive and is only found in trace amounts in the Earth’s crust as a radioactive decay product of 238U.
A BIT OF HISTORY…
Johann Gadolin who was a Finnish chemist, first discovered the oxide mineral he named ‘yttria’ in 1974, and shortly afterwards, ‘ceria’ was extracted by Martin Heinrich Klaproth, Jöns Jakob Berzelius and Wilhelm Hisinger. Subsequently, Carl Gustaf Mosander began work to separate these oxides into their elemental forms (now known as the lanthanides). Indeed, the small Swedish town of Ytterby is now famous for being a main source of the lanthanide elements due to the discoveries of yttrium (Y-a lanthanide analogue), terbium (Tb), erbium (Er) and ytterbium (Yb). The separation of the individual lanthanide elements from their naturally found mineral forms is however, a far from easy task. This is due to the fact that they all commonly exist as the +3 cations in standard aqueous conditions, and they display very similar chemical properties. However, repeated size exclusion type separation techniques (since the size of the lanthanide ions decreases steadily across the periodic series) has proven most useful in separating and therefore isolating individual elements in their pure form for further investigation and use.
LANTHANIDES IN MODERN TECHNOLOGY…
Comprising cerium (element 58) to lutetium (element 71), the lanthanides are unfamiliar to many of us, but they are crucial for everyday technologies from fluorescent lighting to the internet. As a synthetic chemist whose job it is to study the chemistry of the lanthanide ions, I am often asked which one is my favourite. However, this is not an easy question to answer, and my reply depends on which element I have been using in the lab that week! Although a common perception is that lanthanides possess almost identical chemistry
58 59 60 61 62 63 64 65 66 67 68 69 70 71 Ce Pr Nd Pm Sm Eu Gd Tb Dy Ho Er Tm Yb Lu
140.12 140.91 144.25 144.91 150.36 151.96 157.25 158.93 162.50 164.93 167.26 168.93 173.05 174.97
Figure 1. The lanthanides or 4f elements, left to right: cerium, praseodymium, neodymium, promethium, samarium, europium, gadolinium, terbium, dysprosium, holmium, erbium, thulium, ytterbium, lutetium.
REFERENCES:
Element recovery and sustainability: F-block elements recovery
L.S. Natrajan, M.H. Langford-Paden, in RSC Green Chemistry Series, Element Recovery and Sustainability, ch.6, F-block Elements Recovery, The Royal Society of Chemistry, Ed. A. Hunt, (2013) 160-177.
http://www.rsc.org/ shop/books/2013/ 9781849736169.asp
Radiochim. Acta95, 303–311 (2007)/DOI10.1524/ ract.2007.95.6.303©by Oldenbourg Wissenschaftsverlag, MünchenRadiolanthanides in Endo radiotherapy: an overview By F. Rösch* and have in the past been described as boring in a famous quote, each lanthanide (particularly in its cationic form Ln3+) possesses unique physical characteristics. My favourite characteristic is that almost all the 4f elements glow in the dark (or luminesce following light stimulation). Their colours and brightness are similar to those seen in the common glowstick and range from UV, to blue, green, red, yellow, orange and the near infra-red (which our eyes cannot see).
Figure 2. Common emission colours of lanthanides demonstrated with organic fluorescent dyes (red = europium, orange = samarium, yellow = dysprosium, green = terbium, blue = thulium)
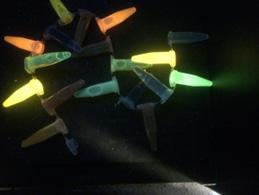
Although the actual chemistry of the lanthanides is quite predictable across the series, e.g. they become more potent Lewis acid catalysts for a range of organic transformations (including polymerisation reactions to make plastics) as the ionic radius decreases due the increasing charge density, they actually display some very intriguing physical properties which are nowadays being commercially marketed. One of the main applications of Gd (III) compounds is as a magnetic resonance contrast agent to aid in the diagnosis of diseased tissue in the human body. Indeed, nowadays, 1 in 3 of clinical MRI scans in hospitals use a Gd (III) contrast agent to enhance scanner images of tumours and blood flow for example. These are most commonly marketed under the trade names DOTAREMTM and MagnevistTM and essentially work by measuring the rate of exchange of water molecules with the Gd (III) ion in a controlled manner, to enhance contrast. The overall experiment is very similar to NMR spectroscopy. Other important medial applications include the use of certain radioisotopes of the lanthanides such as 153Gd, 177Lu and 111Sm for cancer therapy. The lanthanides are perhaps most well renowned for their optical properties (i.e. the fact they glow brightly, with characteristic colours under UVvisible light stimuli (Figure 2). This has resulted in the widespread use of lanthanides in lighting, displays (including white light in incandescent light bulb), the red, blue and green colours in display technologies including iPhones and colour indicators in biological applications and security inks to identify counterfeit money amongst many others. Many lanthanide ions are used in lasers; the main example being Nd in Nd:YAG, (YAG = yttrium aluminium garnet) which can produce bright green light which has been used in medicine, and laser light shows for example. Ytterbium (Yb) is also used in some laser systems and ytterbium fibre laser amplifiers are found in commercial and industrial applications where they are used in marking and engraving. Moreover, erbium finds a niche use in the telecommunications industry and is a vital component in optical fibres for optical digital information transmission including the internet.
Given the majority of the lanthanides in their ionic forms possess a large number of unpaired electrons in their valence orbitals (≤7 unpaired electrons), it is therefore not surprising that they possess unique magnetic properties. In fact, Nd is heavily used in permanent magnets in electric and hybrid electric vehicles (electricity driven bikes, cars and buses), including in windfarms and is one of the strongest magnets known. The remainder of the rare earths find many uses in hybrid electric vehicles, for example, the Toyota Prius and Yaris, the Honda Insight and the Ford Focus Electric, where their uses are varied and range from batteries, lighting, catalytic convertors (cerium), polishing and headlights. In modern day ‘green technologies’ high purity Nd and Dy are utilised in second generation wind turbines that convert wind energy to electrical energy. They also have proposed uses in solar cell technology.
So, what do iPhones, hybrid cars, wind turbines, lasers, Euro bank notes, medical imaging agents and the internet all have in common? Lanthanides of course! So, now when someone asks me which lanthanide is my favourite, I’d have to say all of them as they are all unique and their chemistry is certainly far from boring!