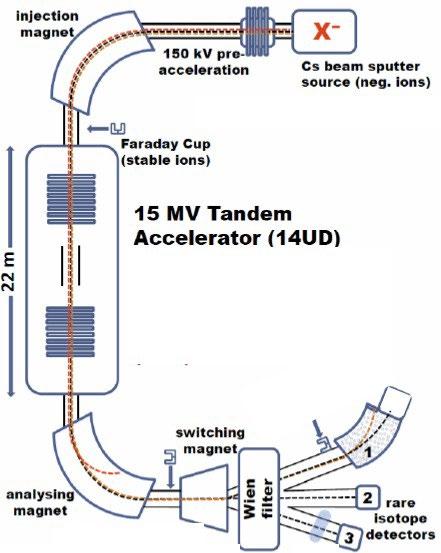
17 minute read
Negative Ion Yield Enhancement for Accelerator Mass Spectrometry of Zirconium-93
Negative Ion Yield Enhancement for Accelerator Mass Spectrometry of Zirconium-93
Harry Bottero – Aurora College
This study investigates different compounds for high yield Accelerator Mass Spectrometry (AMS) of the radioisotope Zirconium-93 (93Zr) by studying temporal behaviour of ion currents of stable 92Zr and 94Zr. The samples were preparedin the AMS laboratory at the Australian National University. Type 1 by mixing powderstogether as solids and types 2 through 4 by dissolving and combining constituent chemicals in concentrated hydrofluoric acid (HF). Silver powder was added to all samples except 10, 15, 20, and 28. The ion current output was monitored in a Faradaycup, and three to four samples of each type were measured. Sample type 2 demonstrated the most efficacious results. There was no correlation between the presence of silver powder (Ag) and the stability and magnitude of the anion beam, northe stability of the isotopic ratio. In conclusion, preparing the samples in solution with the highest ratio of lead fluoride (PbF2) to zirconium tetrafluoride (ZrF4) was the most effective combination for AMS.
Literature Review
AMS is an extremely sensitive scientific analytical technique combining high poweredion accelerators with a mass spectrometer to quantify ratios of a radionuclide to a stable nuclide of the same element. AMS is used to count individual particles with a higher sensitivity by ‘many orders of magnitude’ (Kutschera, 2016) than conventional mass spectrometry. It involves a rare nuclide being separated from all possible background elements with the manipulation of electromagnetic fields after ionization.
Applications of AMS include quantifying minute traces of radioisotopes for astrophysical purposes, ‘past climate studies, ensuring nuclear safeguards’ (Acceleratormass spectrometry, 2021), and organic sample dating by analyzing ratiosof Carbon-14 to Carbon-12. The future of 93Zr analysis by AMS involves two main ideas, progressing nuclear wastemanagement areas and astrophysical models.
The radioisotope 93Zr has a half-life of 1.61 million years (Baglin, 2011). Knowledge on the relative abundance of 93Zr is applicable to nuclear waste management. The radioisotope is an abundant high yield fission product of uranium- 235 (Marks, 2021). Since its halflife is so lengthy, the potency of long-lived radioactivewaste can be quantified with the remaining levels of 93Zr in the decommissioning of nuclear reactors.
A central goal of astrophysics is to model elemental and isotopic abundances in stellarenvironments. The main input parameters for these models are nuclear reaction probabilities. AMS measurements in general and AMS of 93Zr specifically can contribute to determine some reaction probabilities in the lab to improve these models.
However, 93Zr has a stable isobar, Niobium-93, which has virtually the same mass and so acts in the same way in AMS. Being stable means its abundance is many orders ofmagnitude higher than the abundance of 93Zr in any sample material. It poses the mainchallenge for AMS of 93Zr.
The 14UD accelerator at the Australian National University (ANU) is suitable for suppressing background levels of the stable isobar, as the large tandem accelerator terminal operates at up to 14.5MV, the high energies allow for some suppression of the interfering isobar. The 14UD tandem accelerator has been extensively used in theAMS of Iron-60 (Wallner et al., 2020) and Chlorine-36 (Fifield et al., 2013). The hardware of the accelerator is highly tuned for sensitive AMS measurements, thoughis not a dedicated AMS instrument.
The AMS of 93Zr is relatively new and has yet to reach the highest possible sensitivities, due to incursions from background by the 93Nb. The 93Nb background must be further suppressed for more sensitive measurements. Therefore, at the ANU,the purpose of developing methods of 93Zr AMS is to 'achieve the required sensitivity’for its applications. (Pavetich et al., 2019). Chemical element separation techniques inthe preparation of the samples as well as using ‘the right’ chemical compound as sample material can suppress interfering isobars. In 2019, the team were able to lowerthe limit of detection to the order of 1×10-12 by using ZrF4 as sample material and extracting ZrF5- ions to suppress NbF5, which does not readily form negative ions (Carey, 2015).
The drawback is that the negative ion output (ZrF 5 ) is not particularly stable, which has a significant negative impact on the accuracy of AMS measurements of Zr. Addition of lead fluoride (PbF2) and silver (Ag) to the ZrF4 samples increases the negative ion output. To increase stability of the current, stable conditions in the ion source must be reached. To achieve this, more effective chemical mixing methods must be employed for the sample preparation. The optimum mixing ratio of ZrF4, PbF2 and Ag is largely unknown. This investigation aims to draw general conclusions in a trial to determine an indication of trends of the more effective mixing method and mixing ratio.
For future more precise and sensitive AMS measurements of 93Zr, stable ion source output on the timescale of 1 hour per sample is desirable.
Scientific Research Question
What is the optimum mixing ratio of chemical constituents to enhance ion source performance, what is the best way to mix the constituents together, and does it have any effect on performance?
Scientific Hypothesis
Samples produced by mixing Zirconium and Lead compounds in an aqueous chemical synthesis process eventually forming ZrF4 and PbF2 will produce a more stable and prolific anion output than samples prepared by mixing ZrF4 and PbF2 powder. Addition of Ag-powder will enhance output and performance.
Methodology
Experimental Design
The ANU staff prepared the four sample types for the study (Table 1). Four samples were mixed as powders. The rest were mixed in acidic aqueous solution to ensure greater homogeneity of the chemicals. The three aqueous sample types were each individually produced from a single solution. The samples from type 1 were produced individually. Silver was also added individually, and the ratio varies within a sample type. The second preparation method was split into three sample types, with differing ratios of ZrF4:PbF2 (Table 1). Given time constraints, only the samples in Table 1 were able to be tested from the total 31 prepared.
The experimental setup is detailed below in figure one. The samples were placed into the Cathode, and cesium gas, thermally ionized at 1500°C, is accelerated by an electric potential onto each sample. The bombardment of cations 'sputters' material out of the cathode. The material ejected includes many different polyatomic ions, such as ZrF5-. The anions are repelled by the cathode into the pre-acceleration section, which accelerates the ions with an electric potential of 150kV. Then the injection magnet selects the desired mass-to-charge-ratio. The anion beam is then quantified by a Faraday cup.

Figure 1. Schematic of the AMS setup at the ANU. For this study it was used up to the first Faraday cup after the injection magnet. Credits to the AMS group at the ANU for producing the image.
The negative ion output was determined by quantifying the 92ZrF5- and 94ZrF5- beam currents in the Faraday cup after the injection magnet. The stable isotopes 92Zr and 94Zr both have roughly 17% natural abundance. When determining the 93Zr content of samples, which will typically be 109 -1012 less abundant than the stable Zr isotopes the accelerator, additional electromagnetic filters and a particle detector need to be utilized (Pavetich et al., 2019). This is not required nor practical for the presented tests. Using the tandem accelerator was not beneficial for these tests since variations in the accelerating voltage could mimic an erratic ion source behaviour.
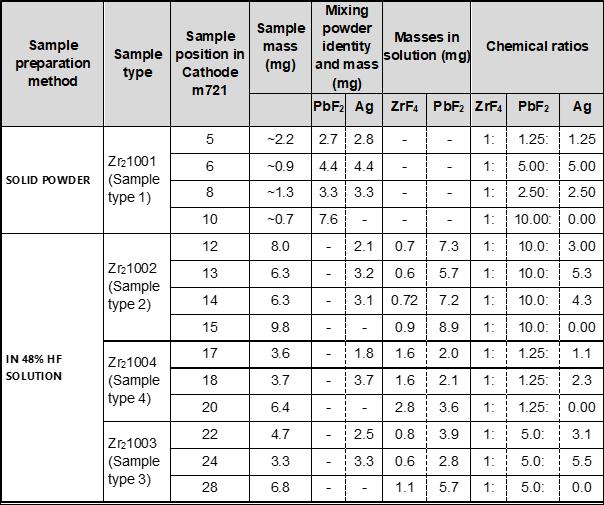
Table 1. Sample Preparation Method and Type Chemical Composition of Each Sample. Details of the samples including their types, the sample preparation method used, the relevant sample masses and the powder mass, as well as the chemical ratios adjusted to ZrF4.
Data Collection
Each test took approximately 65 minutes corresponding to 120 loops. Here, a loop is defined measuring the 92ZrF5 - current for 10 seconds, switch the magnet to 94ZrF5 - andmeasure this current for 10 seconds and then switch back to 92ZrF5 (total of 32.8 seconds). The inclusion of two stable isotopes gives an additional metric, the ratio between the two isotopes aids in indicating sample stability. Due to the sample
physically changing when being sputtered, readjustments of beam focusing is neededoccasionally. ‘Tuning loops’ where this adjustment was performed were excluded. Sample 6 was stopped after 91 loops, because the output was insignificant.
The raw data was retrieved from the system and included into an excel document foranalysis.
Data Analysis
The accelerator’s outputted data was the collected ion current for both isotopes, as well as the isotopic ratio for each loop. From there, the total charge and hence total number of particles were calculated in accordance with the quantification of time generated from the initial ten tuning loops.
To compare between samples and sample types, three factors are used to describe the sample output and stability:
The total number of particles, which is the current integrated over time. A highertotal number of particles for the radioisotope tests reduces the margins of errorand increases the sensitivity.
The time that the sample outputs a constant current from the beginning of the test reflects stability of the ion source. Low values indicate the current droppedor was too unstable.
Finally, the average standard deviation of the mean of the isotopic ratioindicates how the ratios between isotopes varied within the test, which also indicates stability at the ion source.
Performing a statistical test was inappropriate for the data collected, as the continuousvariable tests assume the dependent variable fits a normal distribution, which this datadoes not. Also, the number of repetitions of samples was low, as no two samples wereidentical, and no statistical test is valid to run based on the small population size. This investigation is hence regarded as a trial, from which a general indication of trends isto be derived.
Risk assessment
The possible risks of using the AMS setup were discussed. The tandem accelerator was not used in the test, hence the only threat to health was x-ray emissions from theion source, though while the ion source was in operation, all participants were safe in the control room, which is a great distance away from the ion source. Also, evacuation procedures wherein all involved participants are evacuated to an outdoor meeting point were prepared for.
Ethics Statement This research was conducted under the direct supervision of Postdoctoral Fellow Dr Stefan Pavetich and PhD Student Dominik Koll from the Australian National University(ANU). It was conducted at the Heavy Ion Accelerator Facility (HIAF) at the ANU, andhence was enacted in accordance with their governmentally mandated safety regulations. Permission from the ANU staff was granted before the data was given foranalysis.
Results
The samples prepared as powder (type 1) generally have lower stable times, lower total output, and greater overall isotopic ratio variance. The samples prepared in solution demonstrated higher stable times, higher mean total particles, and smaller isotopic ratio variance. The summary statistics are displayed in Table 2.
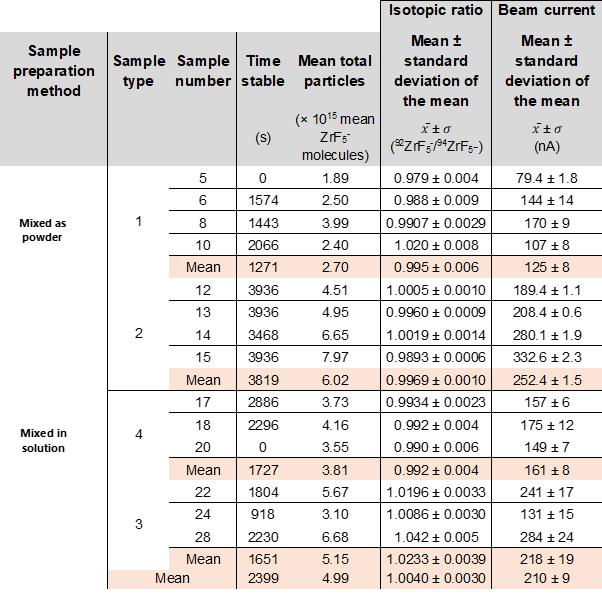
Table 2. Results Summary of Data. Includes the three indicators of sample efficacy, along with the mean isotopic ratio and detector current details, which support quantification of the stability of both outcomes, as well as the strength of maintained current. Both the total particles and detector current is an average of the two isotopes, to illustrate the general current tendencies.
The aqueous samples, specifically type 2 and individual sample 15, displayed the most constant current and isotopic ratio, outputted the highest total amount of particles, and remained stable for the entire time. Therefore, the total number of molecules plot is virtually linear, and the current fluctuates minimally in Figure 2.
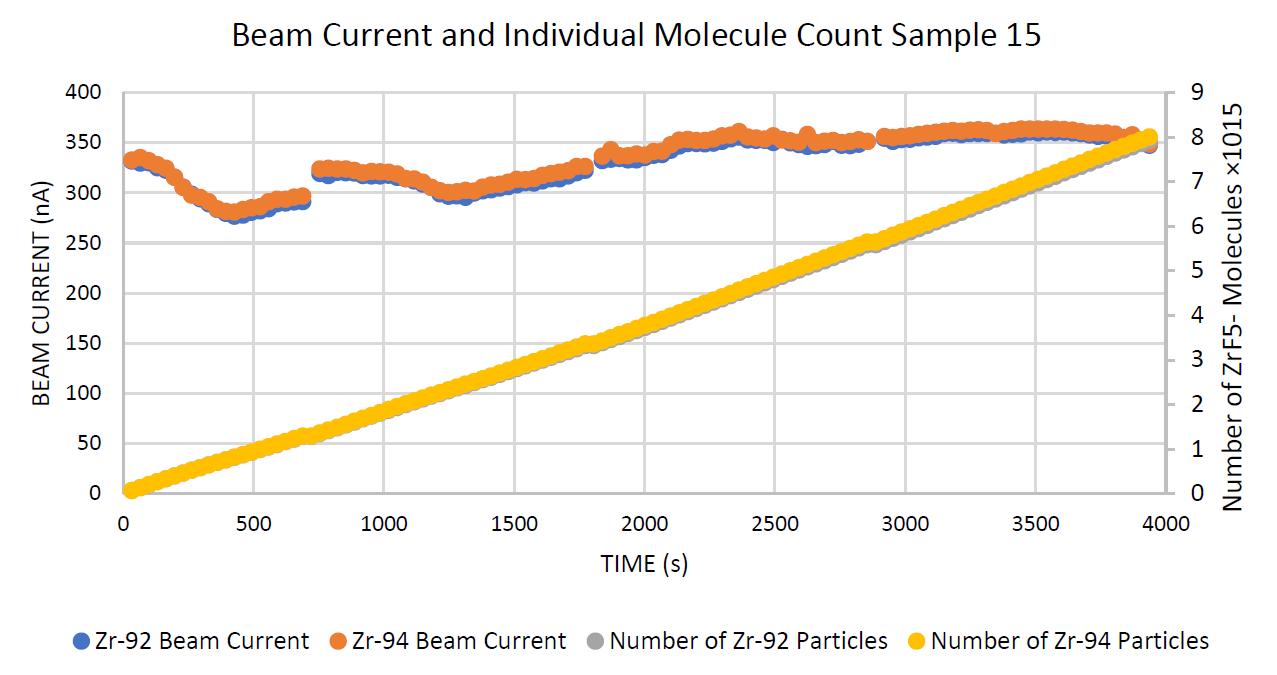
Figure 2. Sample 15, demonstrating an example of a stable sample with a stable current output, in terms of current and molecular count. This sample was the most stable and outputted the highest number of particles. The interruptions in the current curves are the loops where the beam focusing was retuned.
The solid samples, specifically type 1, and individual sample 6, displayed the least constant current and isotopic ratio, outputted the second least total amount of particles, and remained stable for the 5th least amount of time. The sample was stopped after 91 loops, as previously discussed. The total number of molecules only grows insignificantly after the first approximately 1800 seconds, given the negligible beam current. The current fluctuates greatly (Figure 3 & 4)
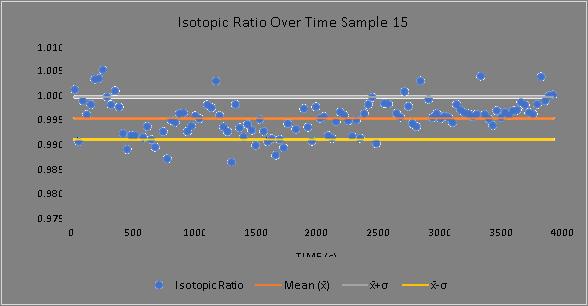
Figure 3. Sample 15, demonstrating an example of a stable sample with a stable current output, in terms of isotopic ratio variance. This sample was the most stable and demonstrated the lowest ratio variance.
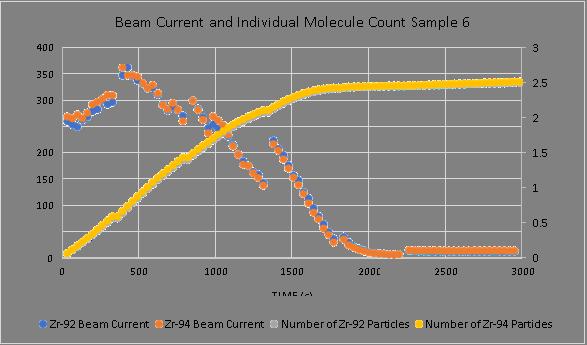
Figure 4. Sample 6, demonstrating an example of an unstable sample, in terms of current and molecular count. This sample was the least stable and outputted the lowest number of particles
Figure 6 shows sample type 2 remained the most constant of the three sample types, beginning with a similar current to types 1 and 4, though sustaining the current for the entire120 loops. Sample type 3 began with a large output of current, though quickly decreased in magnitude. Sample type 1 decreased the most expeditiously.
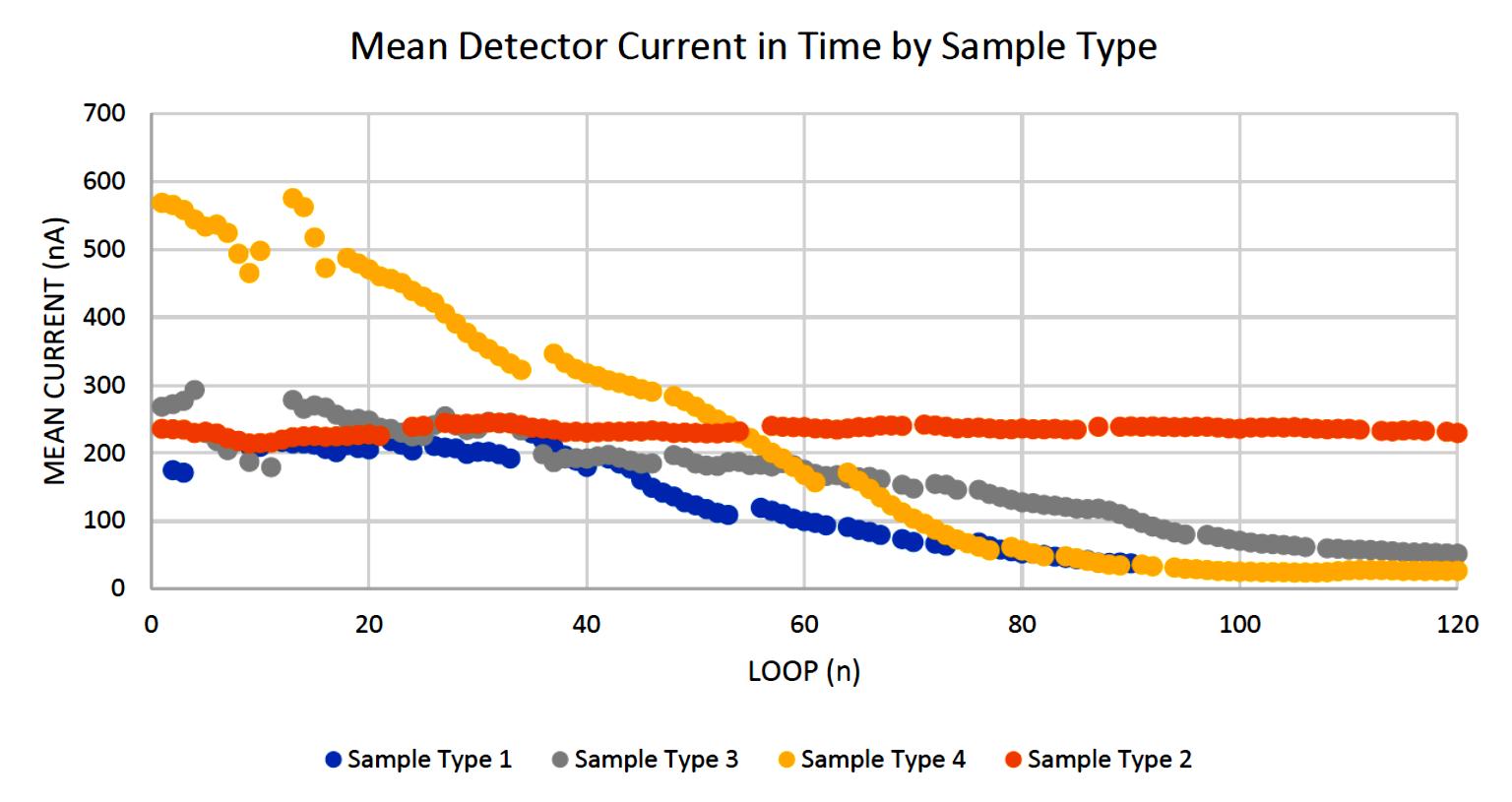
Figure 6. Contrasting the sample types' mean ability to maintain a constant current, taking the mean of all constituent samples of a sample type, and removing all tuning loops. Shows the relative inconsistencies of sample 1, 4, and 3 to sample 2
Figure 7 shows the 92Zr/94Zr ratio for sample type 1 varied the most throughout the loops. The ratio for sample type 2 remained the most constant and was closest to 1. For sample types 3 and 4 there was a similar large variance, and sample type 4 demonstrated an isotopic ratio furthest from 1.
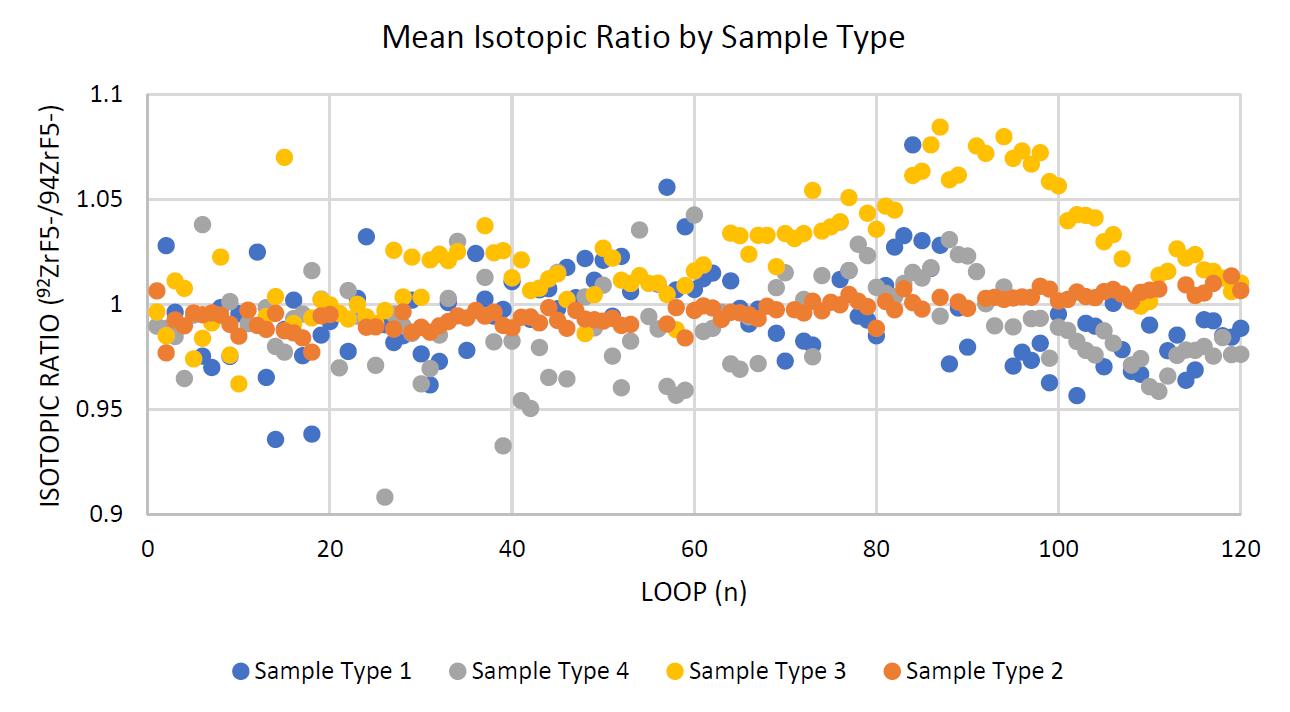
Figure 7. Contrasting the sample types' mean ability to maintain a constant isotopic ratio, taking the mean of all constituent samples of a sample type, and removing all tuning loops. This process introduced many inconsistencies in the smoothness of the data, as illustrated with large variance of data points.
The standard deviation of the mean of each sample ratio is represented in Figure 8. This shows that sample type 1 varied greatly, and sample type 2 consistently varied the least
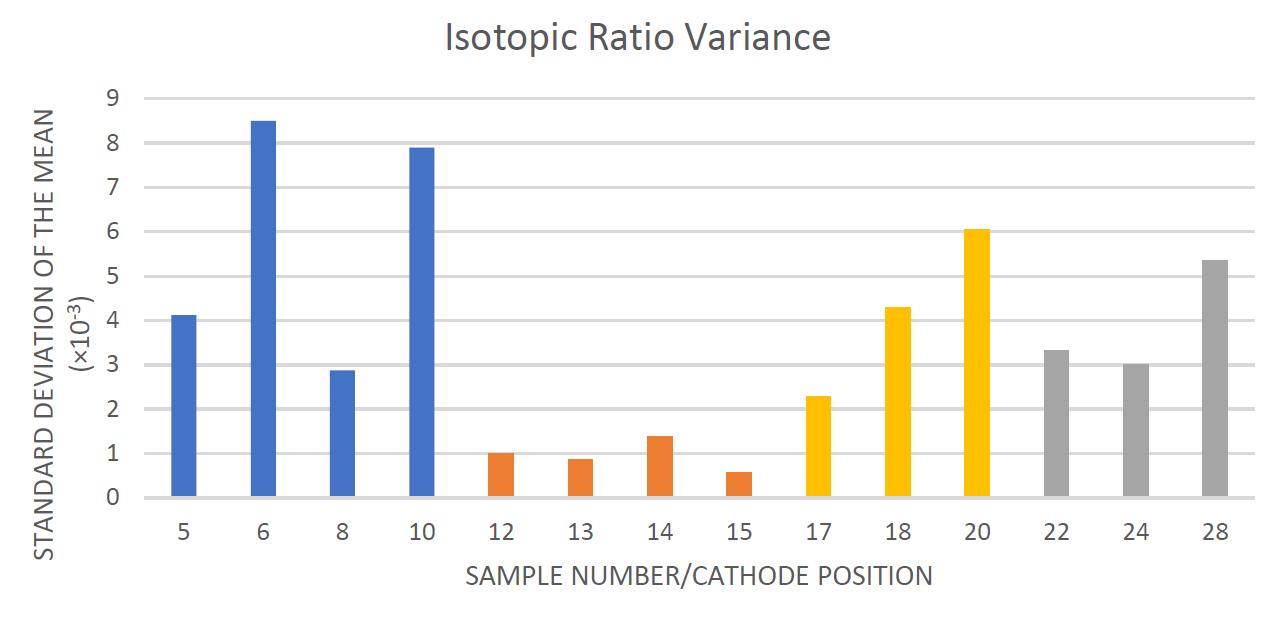
Figure 8. Showing the variances of the isotopic ratio per sample, colour coded for sample types. The bars are colour coded in accordance with their sample type in the same colours as the previous figures.
The time each sample outputted a stable current for is shown in Figure 9. Sample 1 and 3 each had a sample that was never stable, and sample type 2 clearly was consistently stable the entire time for almost all samples.
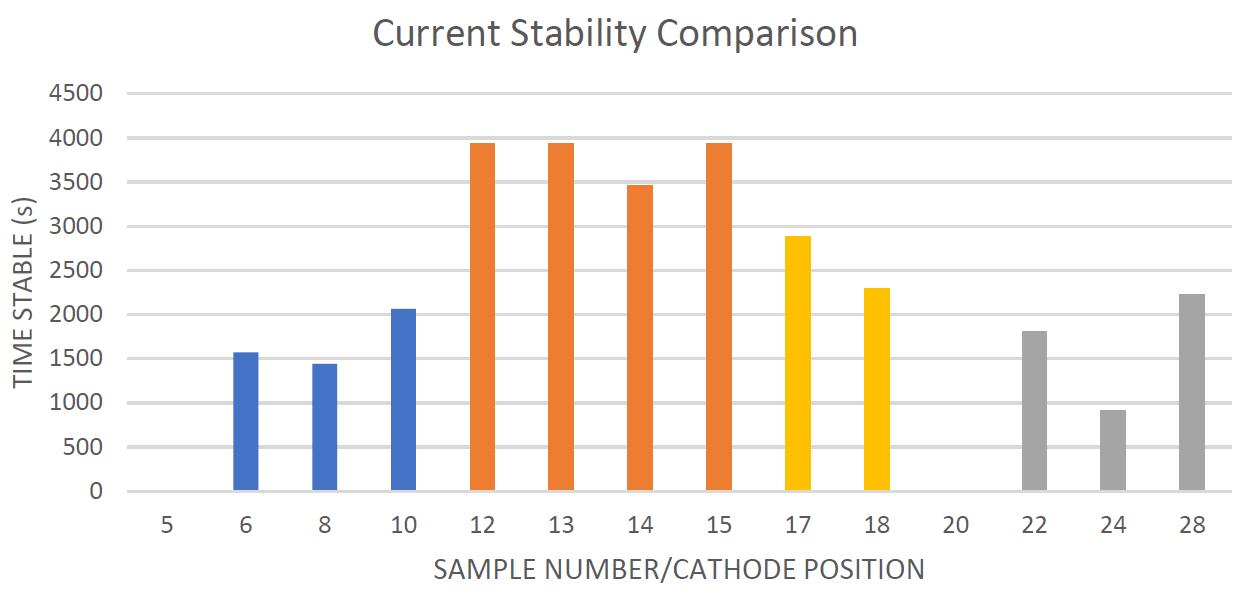
Figure 9. The time each sample remained stable from the start of testing to the first sharp drop. The bars are colour coded in accordance with their sample type in the same colours as the previous figures
Discussion
The aim of this project was to investigate different compounds for high yield AMS 93Zrby studying temporal behaviour of ion currents of stable 92Zr and 94Zr, and to draw conclusions on the general trends of sample composition ratios used in sample synthesis.
As explained in the data analysis section, for a sample to be considered efficacious itmust maintain for the longest time possible a stable and large current and a stable isotopic ratio, as these factors are indicative of sample types which will create the highest possible sensitivity, precision, and accuracy for AMS of 93Zr.
As predicted, the sample types mixed in solution with hydrofluoric acid demonstrateda more desirable, stable, and higher output over a longer procession of loops than those mixed as solid powders. The samples mixed as powder in sample type 1 demonstrated lower and lesser desirable results. This is likely because as the constituent chemicals were combined as solids, the ionic lattices were not dissociated and hence a less integrated synthesis of molecules within the samples was achieved.
This is reflected in the current output fluctuations (Figure 8) as the sputtering encountered different layers of chemicals with higher or lower abundances of constituent chemicals. The chemicals combined in solution dissociated, which allowed a greater miscibility within solution and hence greater homogeneity of chemical constituents within the samples.
Of the sample types mixed in solution; sample type 2 clearly demonstrated the highest mean total particles (Table 2) by sustaining a moderate current for the entirety of the loops tested (Figure 7) with exceedingly high time stable values (Figure 9), and an especially stable mean current (Figure 6). In addition, the isotopic ratio of the sample type was very consistent (Figures 3 and 7) with the variance being the lowest of all samples (Table 2). These results are the most ideal of the sample types, and the mean isotopic ratio ZrF4:PbF2 was approximately 1:10.
Sample type 3 started with an exceptionally large current and quickly decreased (Figure 6). This meant while the mean total particle count was high for the sample type (Table 2), it had large variances. The instability was also evident in the isotopic ratio metrics, wherein the mean variance was close to sample type 4, which performed markedly worse. The sample type was composed of samples with a mean isotopic ratio ZrF4:PbF2 approximately 1:5.
Of the aqueous samples, sample type 4 demonstrated the lowest mean total particles (Table 2) by the current quickly falling and not returning for the entirety of the loops tested (Figure 7) with exceptionally low time stable values (Figure 9), and an especially instable mean current (Figure 6). In addition, the isotopic ratio of the sample type was very inconsistent (Figures 3 and 7) with the variance being the highest of all aqueous samples (Table 2). These results are the least ideal of the sample types, and the mean isotopic ratio ZrF4:PbF2 was approximately 1:1.25.
The significance of sample 15 outperforming the other samples in sample type 2 with remarkably similar chemical ratios is likely due to uncontrolled variables, such as the total amount of sample material, the geometry of the cathode, and sputtering crater inconsistencies. Therefore, variations are expected. The investigation remains valid, as the tests were conducted in a controlled environment with all random errors possibly eliminated and a strict methodology was followed, in accordance with the safety and scientific efficacy policies of ANU.
There was no significant correlation found between any of the variables detailed in the results table to the ratio of silver powder to ZrF5- molecules, nor the masses of silver powder themselves. However more testing outside the scope of this study must be undertaken to determine the extent of correlation more conclusively.
There is a clear positive correlation between the parts of PbF2 to every part ZrF4 in the sample and the ratio’s success in being stable and having a high current output. The relationship is causal, as the controlled environment of the 14UD eliminated all other variables. It is also clear the mixing method and chemical ratios of Sample 2 was the most indicative of sample success.
This project applies to the future of AMS by aiding to improve analysis of the radioisotope 93Zr, the ANU AMS team is currently working on. They can use the conclusions drawn in this paper to help inform the next step they take in achieving the ‘required sensitivities’ of the 14UD’s AMS of 93Zr capabilities for ‘applications in nuclear astrophysics’ and nuclear waste management (Pavetich et al., 2019).
Conclusion
The present study aimed to determine which of the four sample types prepared for AMS of 93Zr would perform most effectively, by maintaining a stable current for the longest time possible, sputtering the maximum number of individual molecules of ZrF5- and showing the least variance in the isotope ratio and current output. It was found that sample type 2 clearly performed most desirably, which was mixed in solution and had a mean ratio of approximately 1:10 of ZrF4 to PbF2. Generally, the samples mixed in solution performed better, and increasing the amount of PbF2 relative to ZrF4 gave a higher and more consistent anion beam output. Therefore, there is a positive correlation between the parts of PbF2 to every part ZrF4 in the sample and the ratio’s success in outputting a high and stable anion beam.
References
Accelerator mass spectrometry. (2021). Retrieved from ANSTO: https://www.ansto.gov.au/acceleratormass-spectrometry
Baglin, M. C. (2011). Nuclear Data Sheets for A = 93. Nuclear Data Sheets, 112, 1163-1389. doi:https://doi.org/10.1016/j.nds.2011.04. 001
Carey, A. (2015). Refining Methods for Ultra-Sensitive Detection of Zirtconium93.Honours Thesis, The Australian National University.
Fifield, L. K., Tims, S. G., Stone, J. O., Argento, D. C., & De Cesare, M. (2010). Ultra-sensitive measurements of 36Cl and 236U at the Australian National University. Nuclear Instruments and Methods in Physics Research B, 268, 858-862. Retrieved from https://doi.org/10.1016/j.nimb.2012.04.02 8
Kutschera, W. (2016). Accelerator mass spectrometry: state of the art and perspectives. Advances in Physics, 1(4). Retrieved from https://www.tandfonline.com/doi/full/10.10 80/23746149.2016.1224603
Leckenby, G. (2017). Remodelling a multi-anode ionisation chamber detector for accelerator mass spectrometry of 53Mn. Canberra: Honours Thesis, The Australian National University. Retrieved from https://doi.org/10.25911/5d69077cdccd8
Lu, W., Anderson, T., Bowers, M., Bauder, W., Collon, P., Kutschera, W., . . . Steier, P. (2015). Zr/Nb isobar separation experiment for future 93Zr AMS measurement. Nuclear Instruments and Methods in Physics Research B, 361, 491-495. Retrieved from https://doi.org/10.1016/j.nimb.2015.01.07 1
Marks, A. (2021). Physics of Uranium and Nuclear Energy. (ANSTO, Editor) Retrieved from World Nuclear Association website: https://worldnuclear.org/information-library/nuclearfuel-cycle/introduction/physics-of-nuclearenergy.aspx
Pavetich, S., Carey, A., Fifield, L., Froehlich, M. B., Halfon, S., Kinast, A., . . . Weissman, L. (2019). 93Zr developments at the Heavy Ion Accelerator Facility at ANU. Nuclear Inst. and Methods in Physics Research B, 438, 77-83. Retrieved from https://openresearchrepository.anu.edu.au/handle/1885/20321 8
Wallner, A., Feige, J., Fifeild, L. K., Froehlich, M. B., Golser, R., Hotchkins, M.A., . . . Tims, S. G. (2020, August 24). 60Fe deposition during the late Pleistocene and the Holocene echoes past supernova activity. Proceedings of the National Academy of Sciences of the United States of America, 36(117), 21873-21879. Retrieved from https://doi.org/10.1073/pnas.1916769117
Winter, M. (2021). Zirconium: isotope data. (U. The University of Sheffield and WebElements Ltd, Editor) Retrieved from WebElements: https://www.webelements.com/zirconium/i sotopes.htm