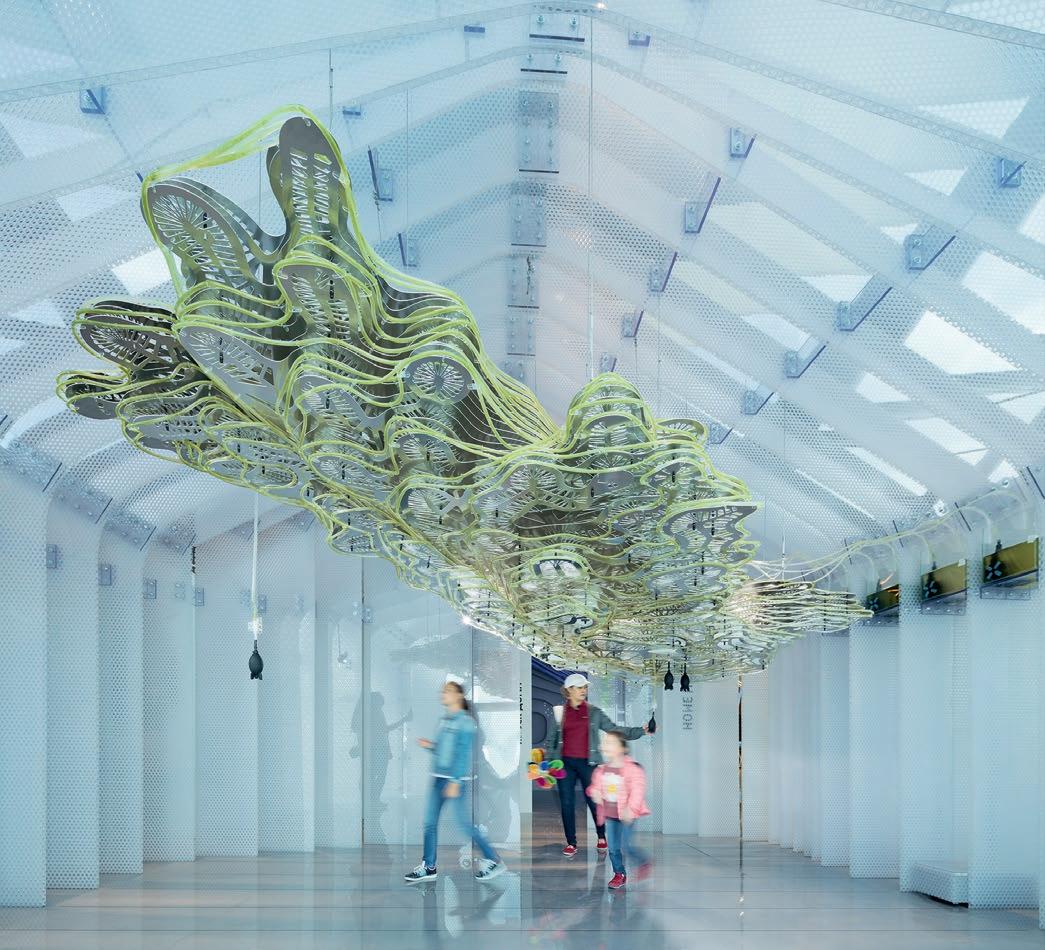
6 minute read
Introduction
Cities emit 70% of global CO2. Buildings alone consume approximately 36% of the world’s primary energy and are responsible for 40% of global CO2 emissions, which is estimated to increase to a further 60% by 2050. According to the Intergovernmental Panel on Climate Change, we must ‘reach “net-zero” climate goal by 2050’ to avert catastrophic impact (Carbon Brief 2019), meaning that any emissions must be balanced by appropriate schemes to offset them.
Algae has the capacity to digest and break down not only CO2 but also other air pollutants such as nitrogen dioxide (NO2) and sulphur dioxide (SO2). Current research indicates that algae also has the potential to capture trace metals dissolved into the environment by biosorption and bioaccumulation processes (Malinska and Zabochnicka-Swiatek 2010). Alongside this, algae can also be harvested to supplement protein intake from animal products, which leads to more sustainable food production and supply chains. The nutritional composition of microalgae is mainly made up of proteins, carbohydrates, lipids and trace nutrients, including A and B vitamins and antioxidants.
This research investigates the materials and conceptual consequences of the integration of microalgae in the built environment. The growth of algae is dependent on the amount of CO2 that it is fed with but also environmental conditions that can be systematically optimised. There are many variables to be considered ranging from habitat and climate to the specific type of algae and the conditions necessary for its growth: solar radiation, temperature and pH. Coupling algal growth with building operations affords a renewed level of efficiency and proposes a novel architectural symbiosis. The projects discussed in this folio can be interpreted as a crucial transition in the architectural paradigm, whereby the urban environment is no longer a container of programmes or functions, as in Le Corbusier’s modernist ideal of ‘a machine for living’ (Le Corbusier 1927), but instead becomes a dynamic process of production, a ‘living machine’.
A total of 13 experimental projects have been carried out with three families being considered as the most significant:
BioTechHut
BioTechHut (6–8) was conceived as a permanent biotechnological dwelling and is composed of three fluidly interconnected environments that loosely embody the fundamental programmes of a living space: the Biolight Room, a dark and calm space in which the only visible light is emitted by bioluminescent bacteria when oxygenated by the air handling system; a further room featuring H.O.R.T.U.S. XL Asthaxantin.g; and a more open environment encompassing the Garden Hut, a space for the production of superfoods and bioenergy. Here, the Algae Photobioreactor Room is filled with growing phototropic micro-organisms that use photosynthesis to generate biomass and oxygen (O2) while absorbing CO2. At the core of the Garden Hut is a harvest area for the processing and transformation of biomass into food and electricity. Measuring 180 m2 in plan, BioTechHut can host a large family and supports 1,600 l of living cyanobacteria cultures in its glass photobioreactors. In optimal conditions, it produces approximately 1 kg of dry algae per day.
5 BioTechHut Astana, 2017. H.O.R.T.U.S. An installation inhabited by photosynthetic colonies of cyanobacteria. Visitors are encouraged to nurture the colonies with CO2 in order to generate O2 and a growing biomass. The living cultures of chlorella growing within the glass photobioreactors can absorb 2 kg of CO2 per day.
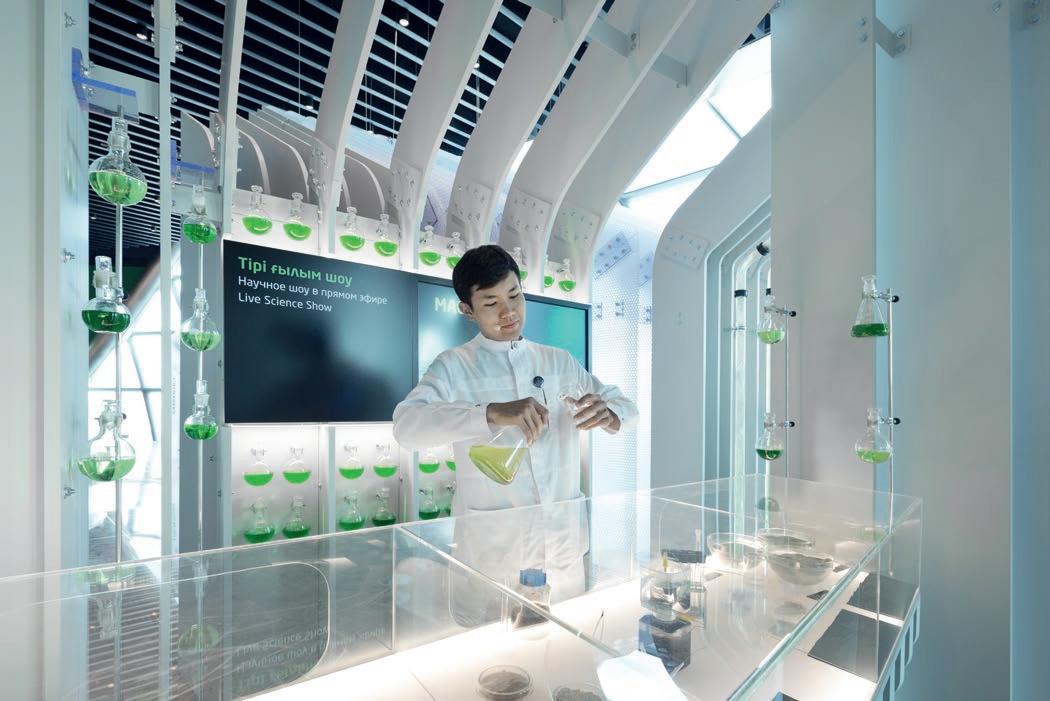
6 BioTechHut Astana, 2017. Garden Hut. Green microalgae can contain up to 60% oil from which 1 kg of biofuel can be produced, releasing 10 kWh of energy, which is enough to power the average home in the UK.
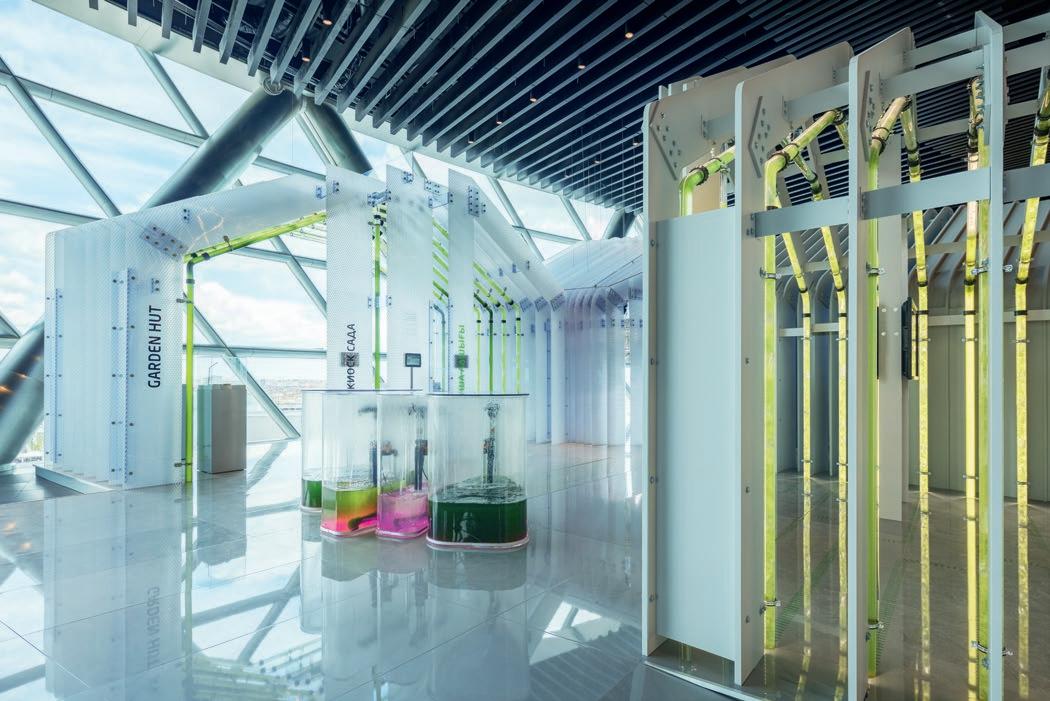
7 BioTechHut Astana, 2017. The Harvest Room. Every day, the BioTechHut produces up to 600 g of protein, enough to supply the recommended daily intake of 12 adults; the equivalent in meat-based proteins from eight cows. 8 (overleaf) BioTechHut Astana, 2017. Glass tubes.
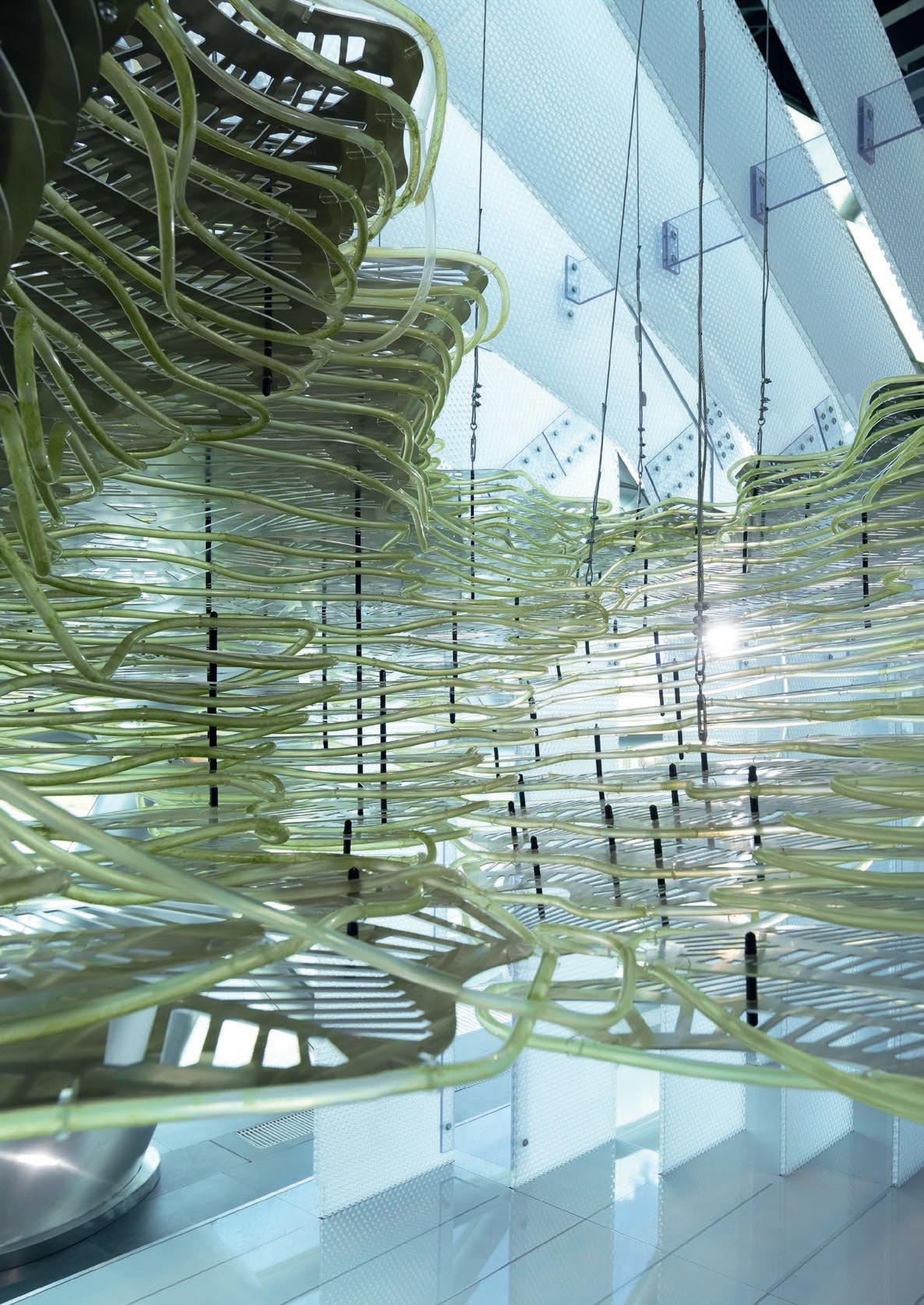
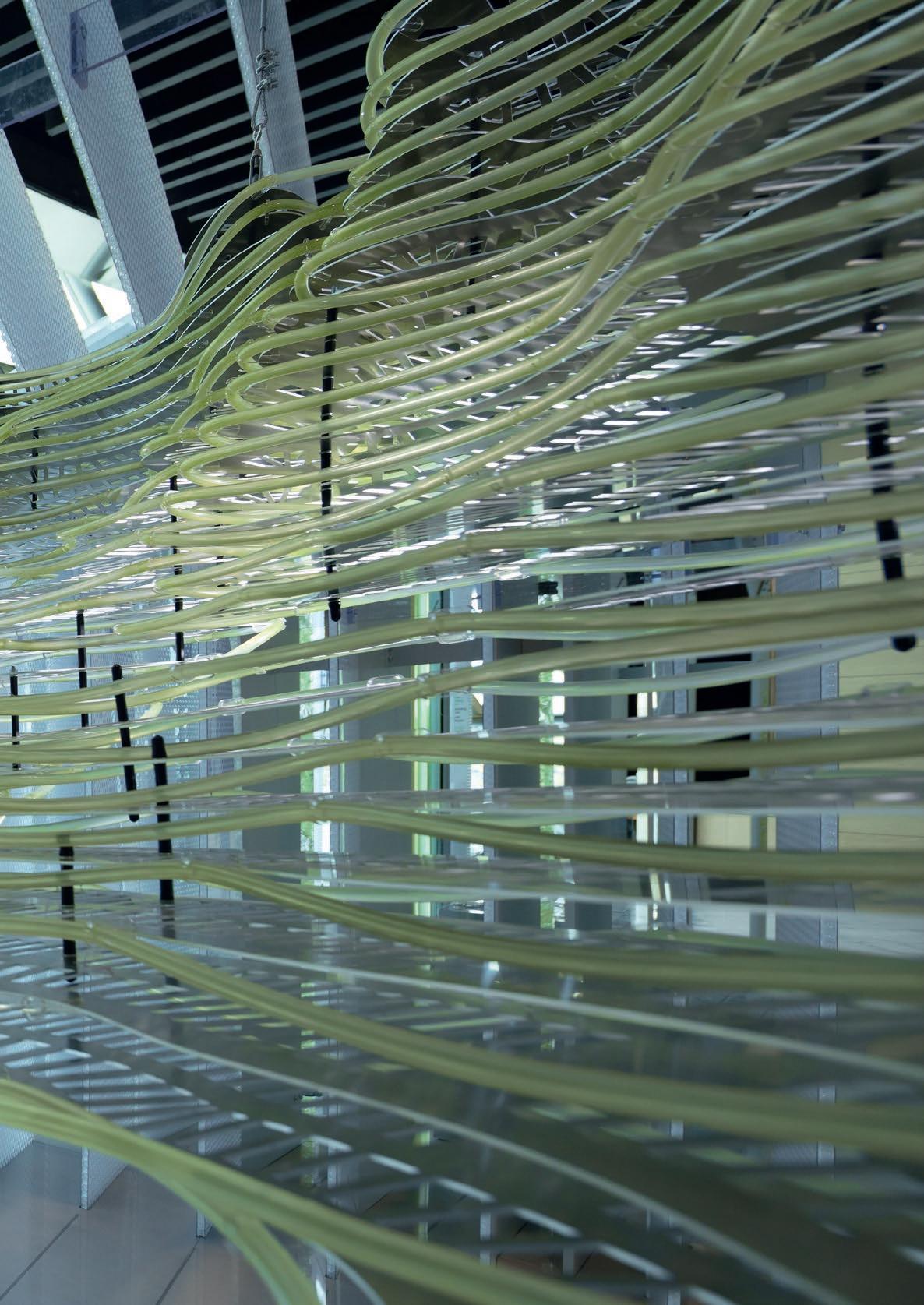
H.O.R.T.U.S.
Hydro Organism Responsive to Urban Stimuli (H.O.R.T.U.S.) is the name of a series (12–3) of photosynthetic sculptures and urban structures that create artificial habitats for cyanobacteria. These structures can also absorb emissions from building systems. They constitute a new active layer of both urban and natural metabolic cycles, thus reconnecting the so-called ‘green’ and ‘dark’ sides of ecology.
H.O.R.T.U.S. XL Astaxanthin.g is inspired by the collective behaviour of coral colonies and their morphogenesis. Individual coral polyps host microalgae called zooxanthellae within their tissues. As the algae photosynthesise, they provide a metabolic flow of energy to the polyps, which in turn use this energy to build their exoskeleton of calcium carbonate. Exposure to sunlight results in more rapid growth. This positive feedback loop enables the characteristic convoluted morphology of many known coral species to emerge (3, 11).
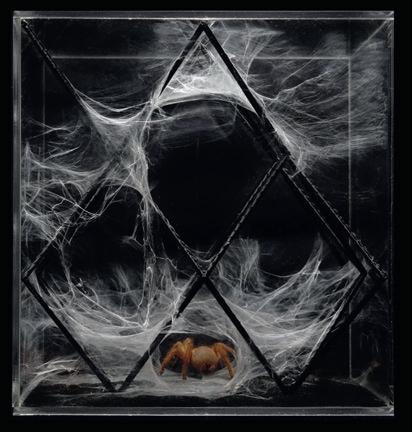
9 XenoDerma, 2019, The Bartlett, UCL. Urban Morphogenesis Lab. Claudia Pasquero with Filippo Nassetti and Emmanouil Zaroukas and with research assistants Meng Xuan Li and Xioa Ling. 10 H.O.R.T.U.S. XL Astaxanthin.g, 2019. Interior view. The metabolisms hosted by the structure are powered by photosynthesis, converting radiation to O2 and biomass. The density of bacteria on each bio-pixel has been digitally computed to ensure the organisms are positioned in areas of increasing incoming radiation.
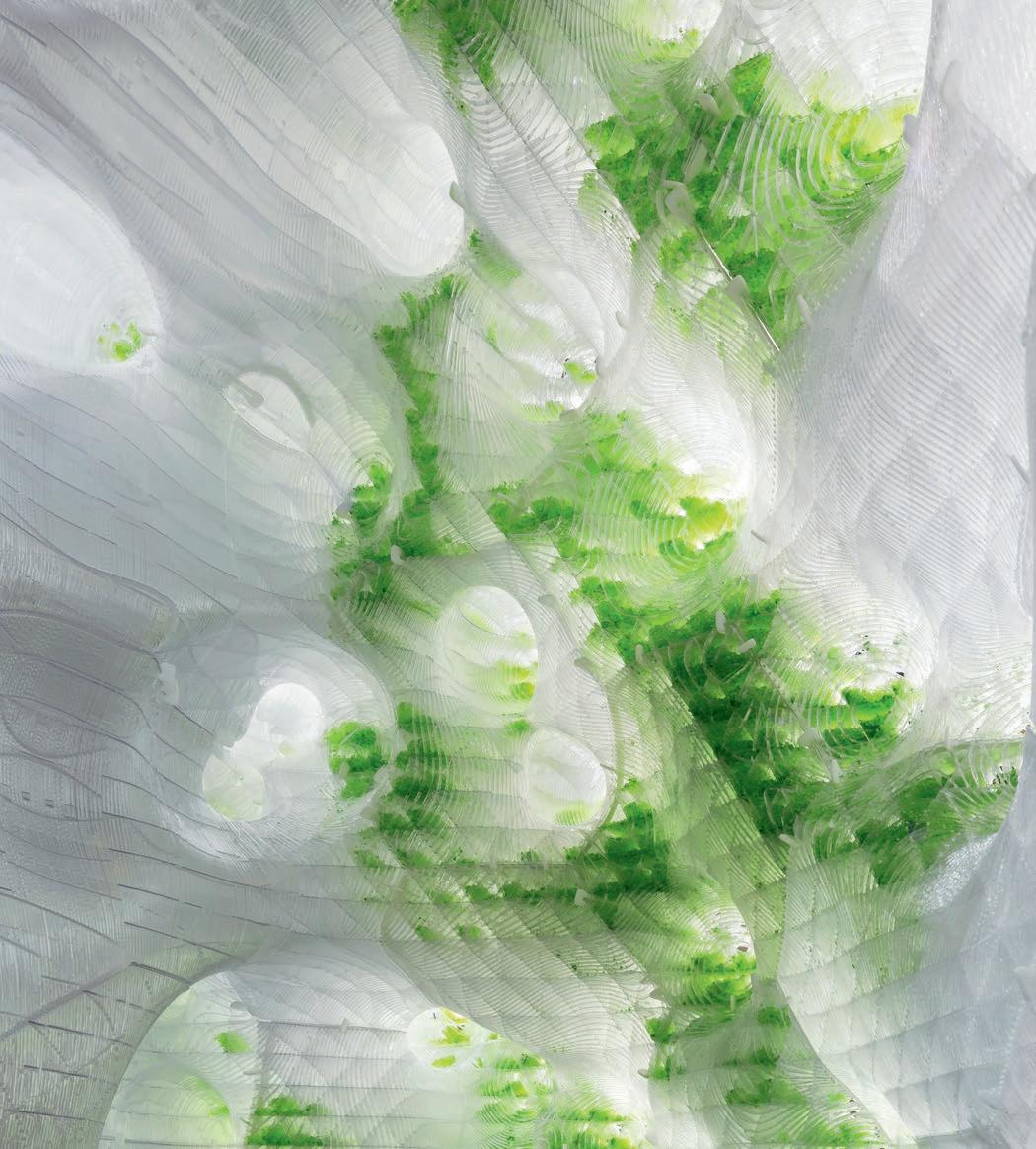
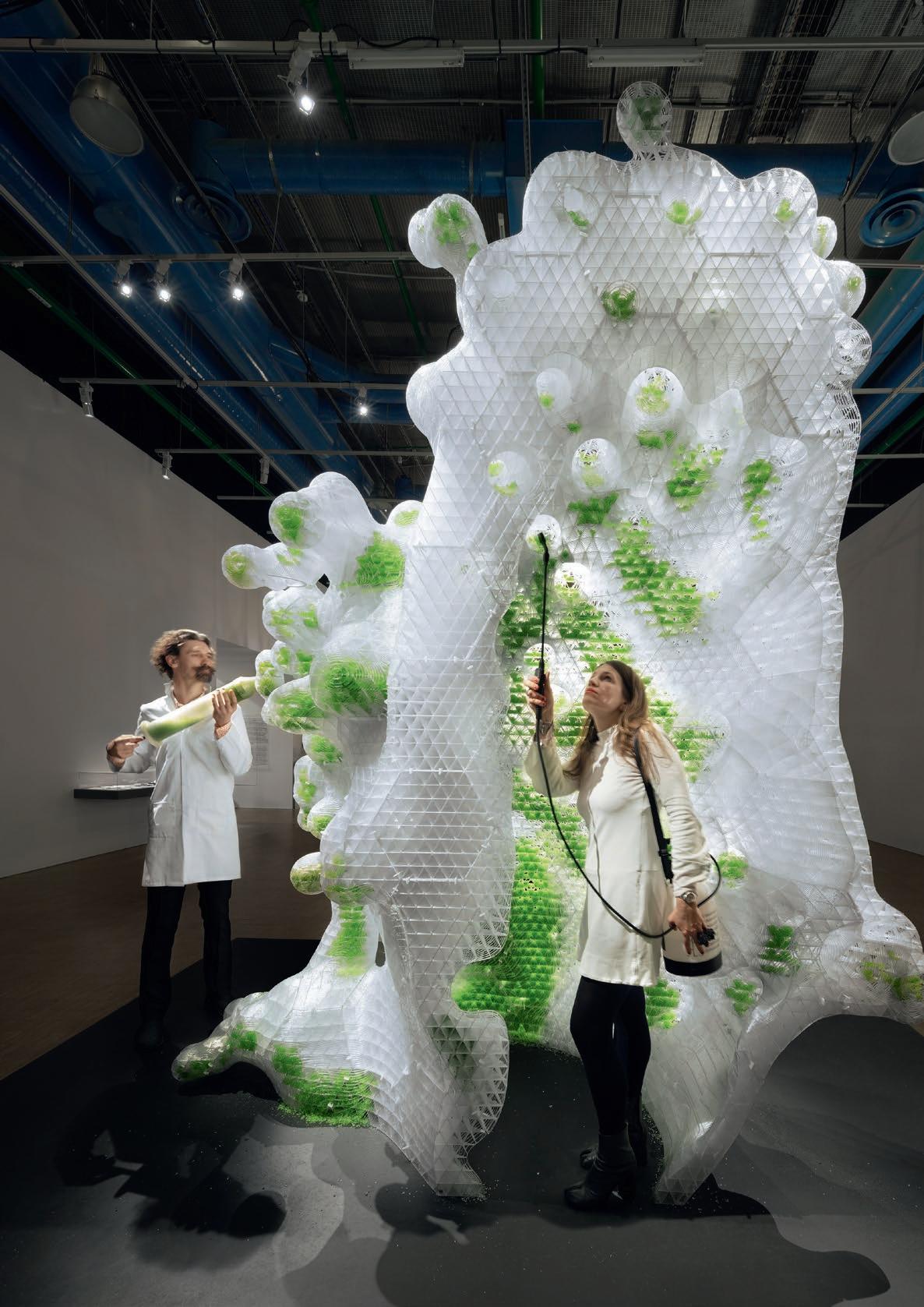
11 H.O.R.T.U.S. XL Astaxanthin.g, 2019. Front view of the installation and cyber-gardeners. A digital algorithm simulates the growth of a substratum inspired by coral morphology, which is digitally deposited by 3D-printing machines. The photosynthetic bacteria are inoculated on a bio-gel medium in triangular units (bio-pixels), arranged to form hexagonal blocks of 18.5 cm.
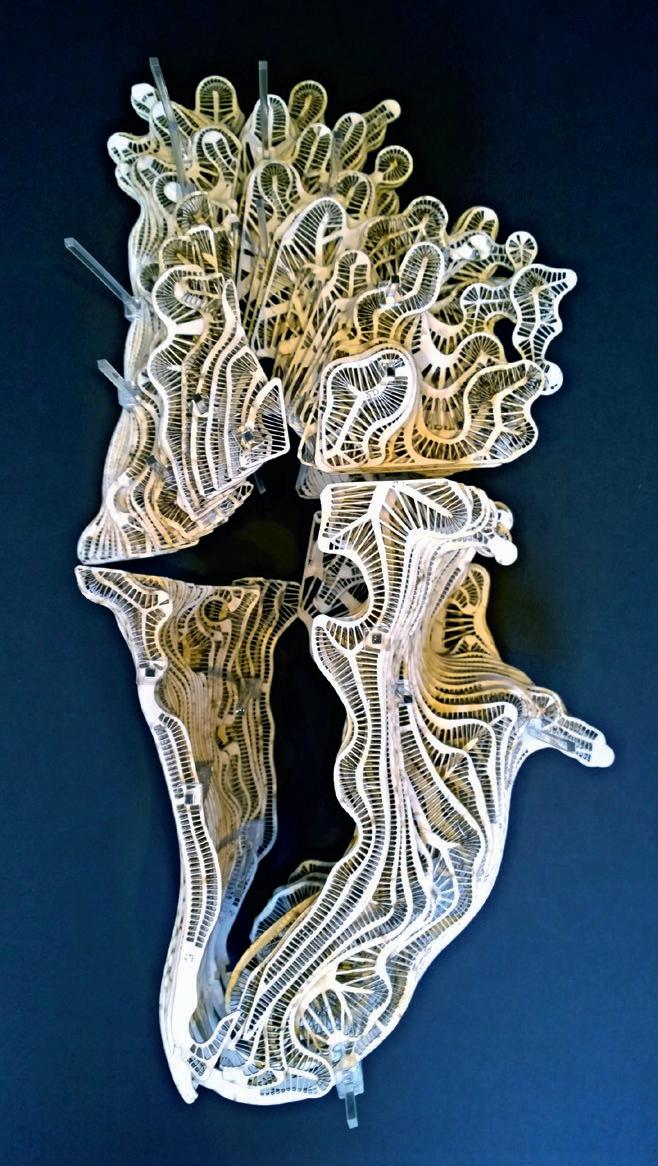
12 12 H.O.R.T.U.S. Astana, 2017. Study 1:10 model.
13 H.O.R.T.U.S. Astana, 2017. Cutting lines plan.
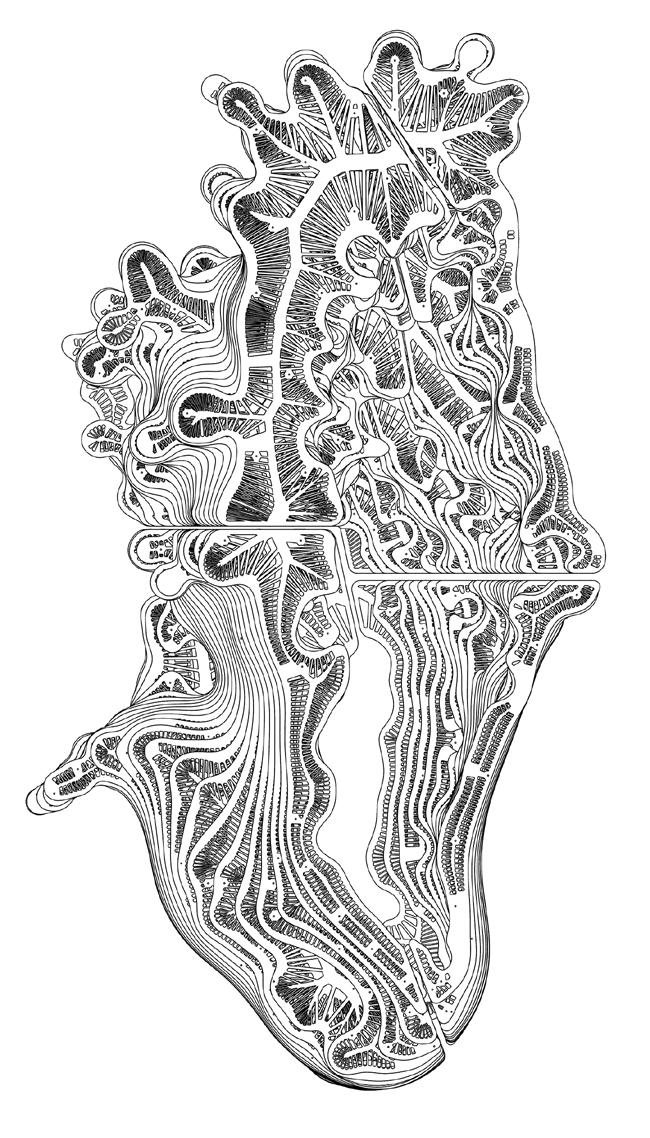
13
PhotoSynthEtica
PhotoSynthEtica Dublin is a large-scale façade installation designed for Climate-KIC, a prominent EU initiative with an aim to accelerate the adoption of nature-based solutions to tackle the global climate crisis. The project was conceived as an ‘urban curtain’ that can capture CO2 from the atmosphere and store it in real-time at a rate of approximately 1 kg of CO2 per day, which is equivalent to that of 20 large trees.
Composed of 16 modules, each 7 × 2 m, this unique curtain prototype enveloped the first and second floor of the main façade of The Printworks at Dublin Castle (15–6). Each module functions as a photobioreactor, a digitally designed and fabricated bioplastic container that utilises daylight to feed the living micro-algal cultures and releases luminescent shades at night. Unfiltered air is introduced at the bottom of the façade and rises through the watery medium within the bioplastic photobioreactor, coming into contact with voracious microbes. CO2 molecules and air pollutants are captured and stored by the algae and grow into biomass. This can then be harvested and employed in the production of raw material for bioplastic products, such as the main building material of the photobioreactor itself. To culminate the process, freshly photosynthesised O2 is released at the top of each façade unit into the urban microclimate.
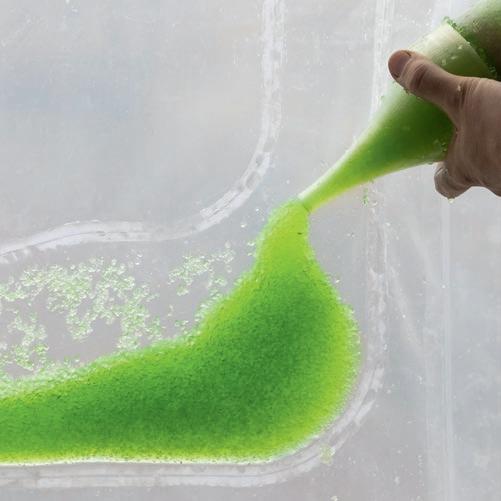
14 PhotoSynthEtica Dublin, 2018. Inoculation detail. Thanks to their serpentine design, the modules optimise the carbon sequestration process.
15–6 (overleaf) PhotoSynthEtica Dublin, 2018. Façade view. The full curtain pattern is reminiscent of a large trading data chart that embodies Climate-KIC’s commitment to solve the global climate crisis.
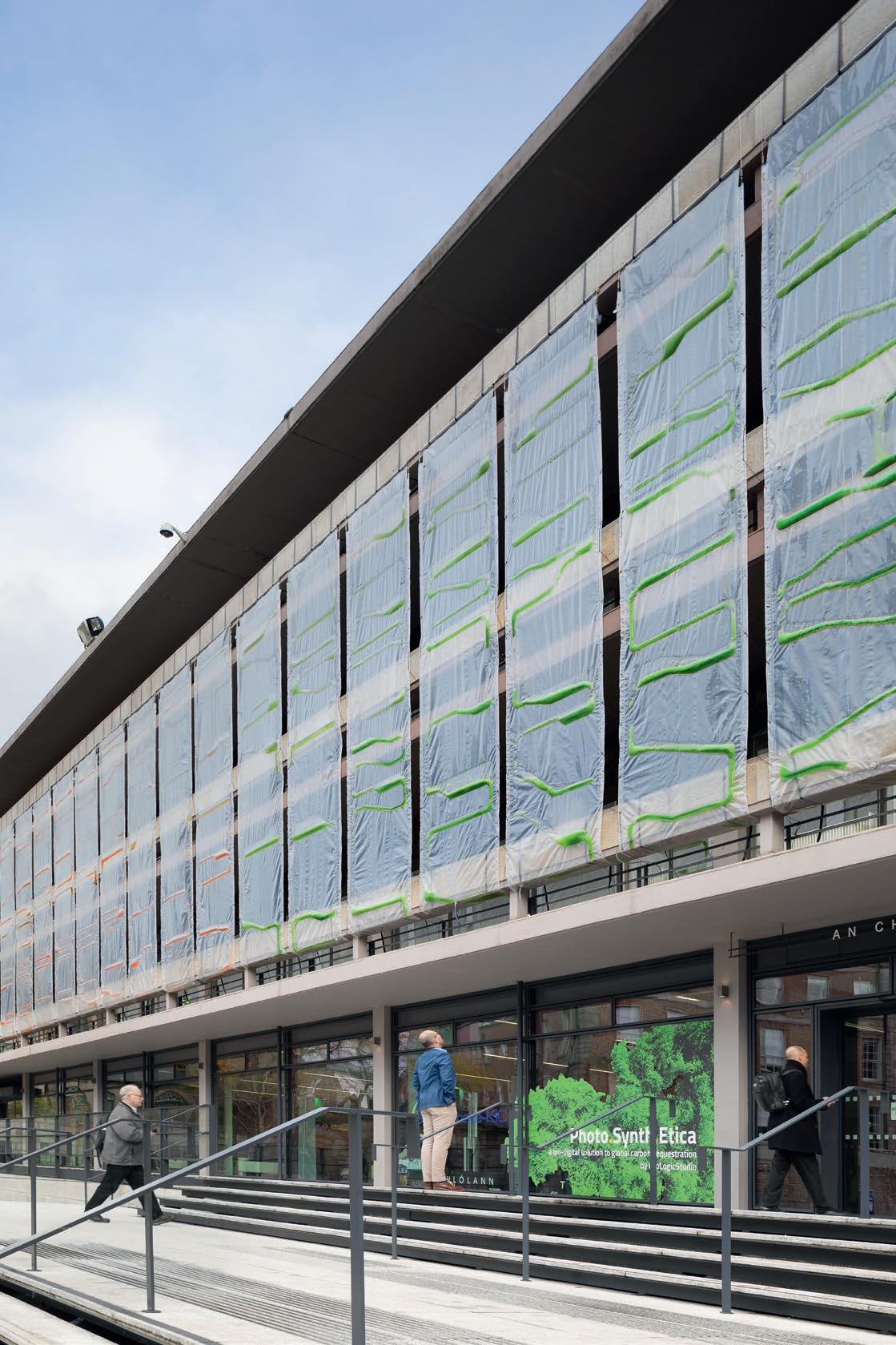
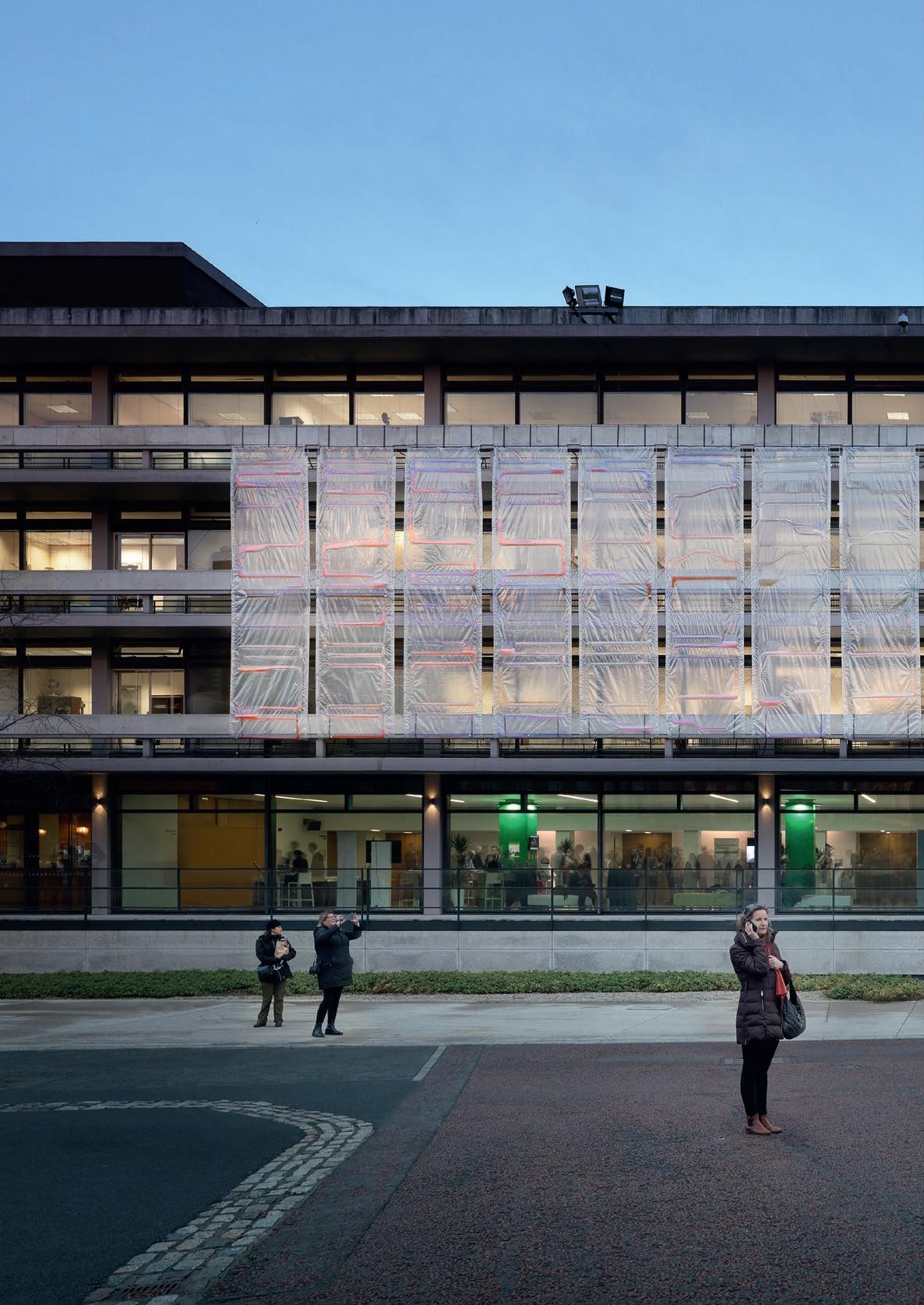
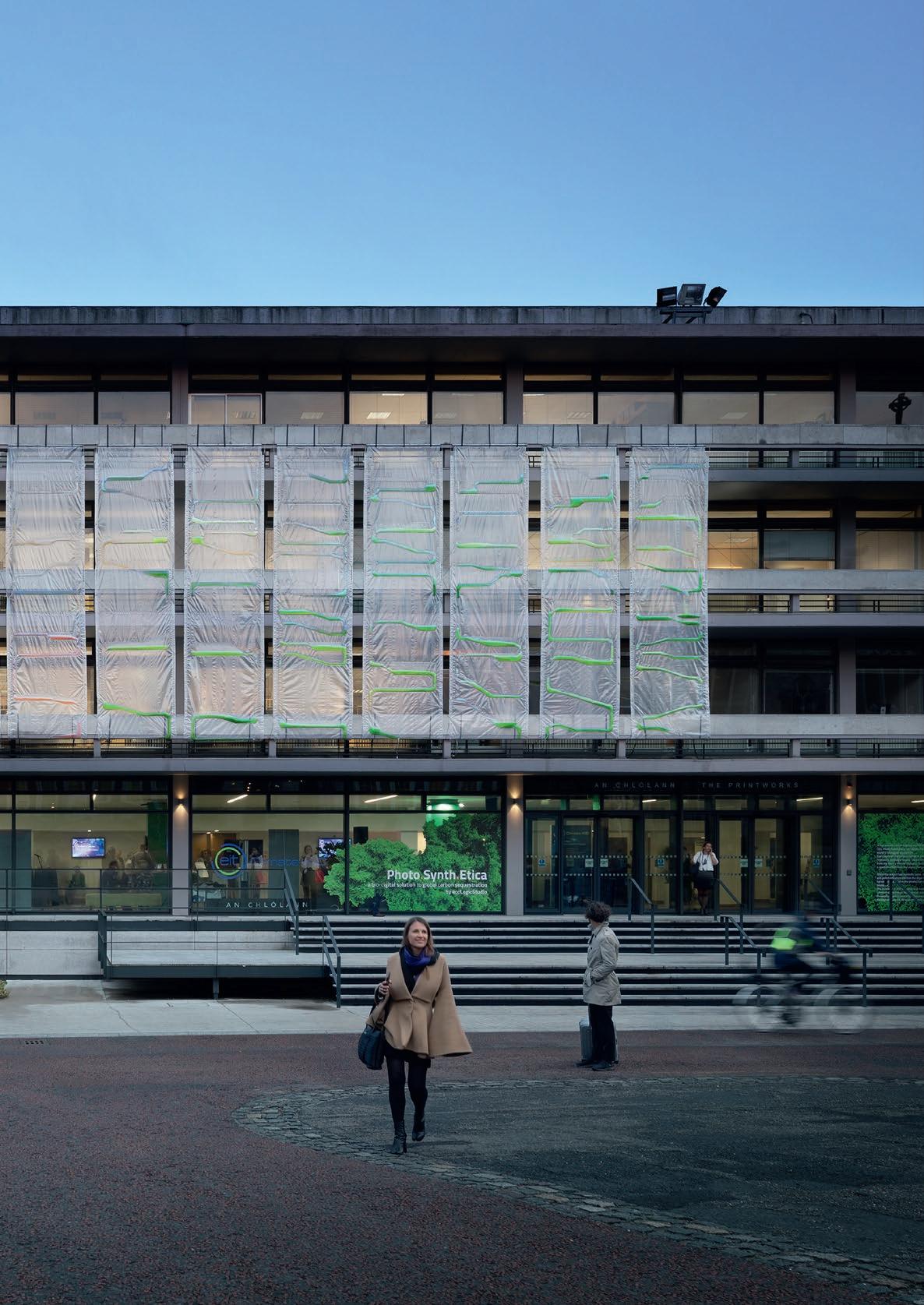