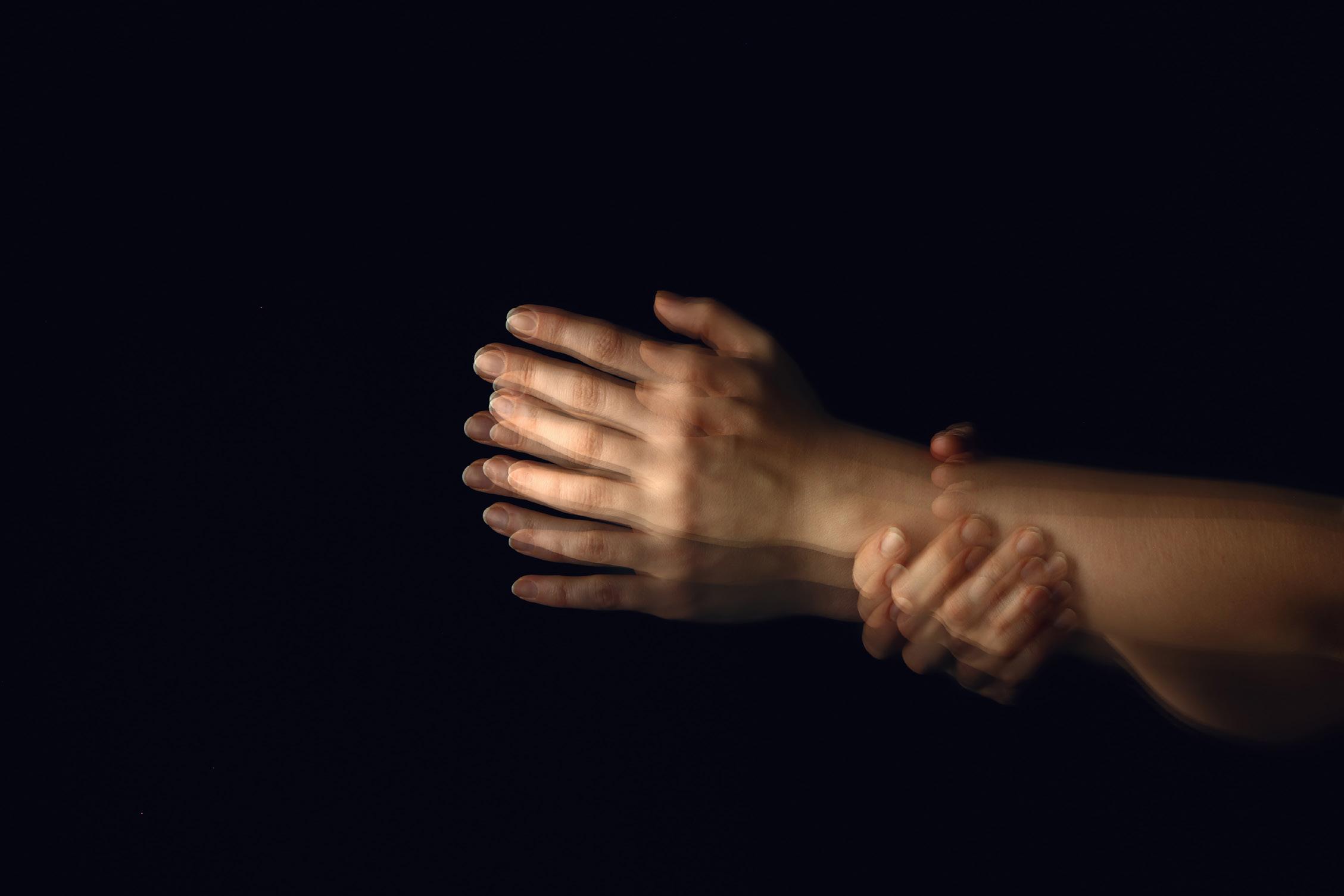
30 minute read
Parkinson's Disease and L-DOPA
Rebecca J. Anderson, PhD
James Parkinson was a colorful character. In addition to following his father as a practicing surgeon in London, James actively pursued geology, paleontology, and politics. In 1807, he became a founding member of the Geological Society of London, along with other notables including Sir Humphry Davy. Parkinson’s geological monograph, Organic Remains of a Former World, remained in print for half a century (1).
Advertisement
Parkinson was possibly the only person ever to win a raffle for a natural history museum. In 1785, Sir Ashton Lever was bankrupted by his wide-ranging collection of natural wonders. Parkinson assumed ownership of the museum and no doubt added his own extensive collection of fossils, until he, too, could no longer finance it. In 1805, the collection was divided into lots and sold (1).
Politically, Parkinson was an advocate for the underprivileged and an outspoken critic of Britain’s conservative government. He engaged in several social and revolutionary causes, was a member of several secret political societies, and wrote 20 pamphlets calling for social reforms and universal suffrage.
In 1794, Parkinson’s involvement with those activities implicated him in a conspiracy called the “Pop-gun Plot.” The plotters planned to shoot King George III in the neck with a poisoned dart while he sat in his box at
But amongst all of his eclectic activities, Parkinson is best remembered for an essay he published in 1817.
Clinical Clarity
At that time, physicians evaluated and attributed individual neurological symptoms to distinctly different diseases (2). Parkinson managed to piece together the medical histories of patients over many years and realized that, first, some neurological symptoms emerged and intensified very gradually. And second, those symptoms were all connected and part of one syndrome (2).
With impressive clarity, Parkinson took the observations of a very limited number of cases and came up with insightful conclusions. He documented all stages of the syndrome in only two patients through detailed medical histories and observed their clinical decline over several years. His remaining four cases were fragmentary. Three of them were men he casually observed while walking along the streets of London. The final case was a man who relocated and was lost to follow up (2).
All six were men over the age of 50 (2). It remains true today that the disease is more common in men than women, and symptoms typically appear in patients over 50 (3).
Parkinson said the initial symptoms were so slight and progressed so slowly that the patients could not recall precisely when the impairment began (2). The first perceptible symptom was a slight weakness and some trembling, usually in one of the hands or arms. After perhaps a year or more, the same symptoms appeared in the other hand and arm.
A few months later, patients developed a stooped posture, which was most prominent while walking. Following this, one of the legs began to tremble and became easily fatigued. A few months after that, the other leg also exhibited trembling and a loss of power. The legs could not rise sufficiently and moved less smoothly, making the patient at risk of falling. The diminished function only hampered fine motor activities, like handwriting. As the disease progressed, writing, eating, and walking became more difficult (2). Progressing further, the arms and legs could not be willfully directed, and the patient could not perform ordinary tasks of daily living. There was also a constant, resting tremor, which stopped temporarily when the patient voluntarily moved. The patient constantly leaned forward and took short, quick, shuffling steps. Eventually, even walking failed to dampen the tremors (2).
The resting tremor became so pronounced that sleep was disturbed and could awaken the patient. Constipation, which was one of the earliest signs, became so severe that powerful laxatives or purgatives were needed (2).
In the final stages of the disease, the trunk was constantly bowed, muscle power was greatly weakened, and the tremors became violent. The patient walked with great difficulty. Chewing and swallowing were also difficult. Bowel and bladder function were involuntary. Death eventually resulted from extreme exhaustion (2).
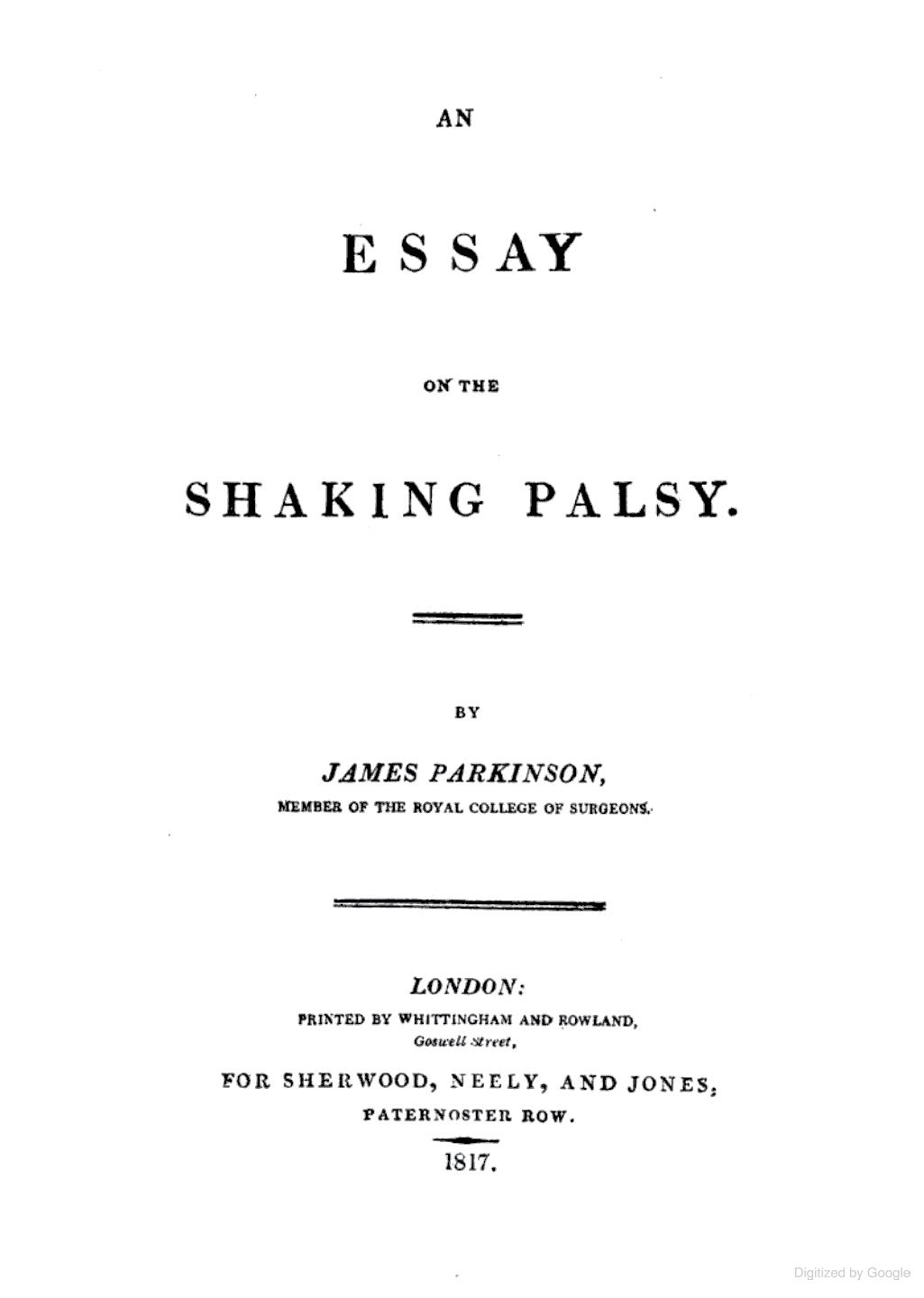
Based on these clinical observations and his understanding of neuroanatomy (about which little was known at the time), Parkinson suggested that this syndrome was a disease of the brainstem and specifically cited the medulla oblongata (2). In that, he was surprisingly close to the actual neuropathology.
Parkinson called this syndrome the shaking palsy. Today, everyone calls it Parkinson’s disease.
Taking the Challenge
An Essay on the Shaking Palsy was published in 1817 (2). Parkinson readily admitted that his conclusions were “immature and imperfect,” but he hoped his essay might inspire others who had the “abilities and opportunities” to conduct more thorough research (2). With a better understanding of the disease and early diagnosis, he said, physicians might be able to effectively treat or even cure it.
Of those who took up the challenge, Jean-Martin Charcot, the most influential neurologist of the 19th century, made the greatest contributions, including coining the term, “Parkinson’s disease.” In the mid-1800s, Charcot and his students at Salpêtrière Hospital in Paris provided a more detailed description of the syndrome, adding to, but not contradicting any of, Parkinson’s observations (4).
William Gowers in London also made notable contributions. Over the next century, other clinicians supplemented descriptions of the disease’s clinical and morphologic progression (4).
The only treatments that James Parkinson offered were the customary remedies of the early 1800s: bloodletting and inducing skin blisters. The first effective therapies were introduced by Charcot and Gowers (4).
Charcot eagerly tried new treatment strategies when the traditional ones failed. But he just as readily rejected those new remedies if they also failed. Anticholinergic drugs were an exception (4).
The 1867 medical thesis of Charcot’s intern, L. Ordenstein, described treating parkinsonian tremor with belladonna alkaloids. Charcot first administered belladonna to patients with Parkinson’s disease in 1892 (4, 5). Of the many centrally acting anticholinergic agents available, he preferred hyoscyamine. This plant-derived compound was usually incorporated into bits of white bread or given as a syrup (4).
Gowers also prescribed hyoscyamine but experimented with arsenic, morphia, hemlock, and cannabis. He specifically noted that the combination of cannabis and opium lessened tremors (4). (Cannabis is known to have some dopaminergic activity.)
Up to the mid-20th century, a wide variety of centrally acting anticholinergic drugs were prescribed. They all had similar efficacy and side effect profiles (4).
Biochemical Pathfinders
At the turn of the 20th century, John Jacob Abel (the founder of ASPET) and Jokishi Takamine, working independently, isolated a hormone from animal adrenal glands, which Abel called “epinephrine” and Takamine called “adrenalin” (6). In 1901, Thomas Aldrich, a chemist at Parke, Davis & Company, crystalized the substance and correctly identified its chemical structure (6). A few years later, chemists synthesized the compound, the first hormone to be John J. Abel, American synthesized in the lab (5). pharmacologist and biochemist, founded Casimir Funk, a Polish chemist who ASPET in 1908. emigrated to London, was interested in how animals produced adrenaline and suspected that it was made from the amino acid, tyrosine (5). Funk’s attempts to prove that theory failed, but despite that, he went ahead and synthesized 3,4-dihydroxyphenylalanine, which he thought might be a biochemical precursor of adrenaline. He presented his results on July 22, 1912, at the Physiological Society of Great Britain (5). Markus Guggenheim, a researcher at HoffmannLaRoche in Switzerland, followed up on Funk’s results. Funk’s compound was racemic. Guggenheim focused on the L-form, because L-enantiomers are the physiologically active form of amino acids (5). Guggenheim performed some pharmacological experiments and found that this L-compound had little
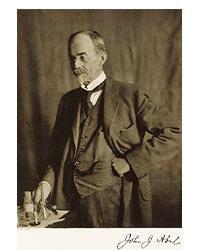
Guggenheim continued his research and gave some of his compounds to his uncle, Bruno Bloch, who was Chief of the Department of Dermatology in the Medical Clinic of Basel County Hospital. Bloch correctly identified Funk’s L-compound as a key intermediate in the conversion of tyrosine to biologically active amines, such as adrenaline (5).
Bloch found the compound’s chemical name cumbersome and shortened it to DOPA, which is an acronym of its German spelling: Dioxyphenylalanin. It was an imperfect choice, because DOPA has a mildly scatological meaning in Polish (5).
In the late 1930s, a decarboxylase enzyme was discovered and helped to explain the stepwise biochemical conversion of tyrosine to epinephrine: tyrosine to L-DOPA, L-DOPA to dopamine, dopamine to norepinephrine, and finally norepinephrine to epinephrine (5, 8). (In 1952, Sir Henry Dale suggested the name dopamine (i.e., the amine derivative of DOPA) because its full chemical name, 3,4-dihydroxyphenylethylamine, was cumbersome.) (8)
Dopamine had been synthesized in 1910 by chemists George Barger and A.J. Ewins at the Wellcome Laboratories in London, and Henry Dale had tested it (9). Dale found that dopamine had only weak epinephrinelike effects on peripheral smooth muscle preparations. Like Guggenheim’s assessment of DOPA, Dale dismissed dopamine as physiologically unimportant (4, 5, 7, 8, 10).
Switching Gears
In the early part of the 20th century, evidence accumulated that synaptic transmission in the peripheral nervous system was mediated by chemicals, primarily acetylcholine and norepinephrine (10, 11). But very little evidence supported chemical transmission in the brain. Most neurophysiologists stuck to the notion that synaptic transmission in the brain was electrical (10).
The research that convinced those neuroscientists that the brain relied on chemical neurotransmission came out of Arvid Carlsson’s desire to get promoted (10).
Even upon entering medical school at the University of Lund, Carlsson aimed to become a researcher (12). World War II temporarily diverted his attention. As he was beginning his clinical training in 1944, he was assigned to examine people who had arrived in Sweden from German concentration camps. Count Folke Bernadotte, a member of the Swedish royal family, had arranged evacuation of 30,000 captives, including 11,000 Jews. Many were children. Carlsson found they suffered from malnutrition and tuberculosis (12).
After that humanitarian diversion, and having passed his pharmacology exams, Carlsson was offered an unpaid research position in Lund’s pharmacology department. In 1951, he received his MD and PhD degrees from the University of Lund and immediately joined the pharmacology faculty (12).
In 1955, the university’s expert committee informed Carlsson that to advance to associate professor, he needed to redirect his research. His studies of calcium metabolism were not considered central to pharmacology (10).
On the advice of colleagues and through several referrals, Carlsson obtained a visiting researcher position under Bernard Brodie, Chief of the Laboratory of Chemical Pharmacology at the US National Heart Institute (7, 10, 11).
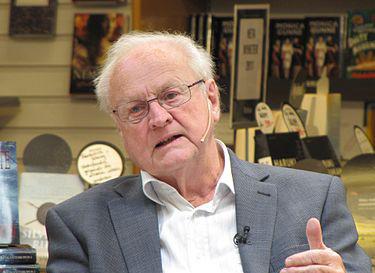
Arvid Carlsson, Swedish neuropharmacologist, was awarded the Nobel Prize in Physiology or Medicine in 2000.
The Role of Reserpine
A shy, introspective teenager, Brodie had become a championship boxer in the Canadian Army, simply (he said) to avoid getting hurt. He was a mediocre student until a chemistry project sparked his interest in organic chemistry (13).
Brodie’s forte was developing methods and general principles for accurately measuring drugs and their
metabolites in biological tissues (13). At NIH, he built a well-deserved reputation in drug metabolism research. Many considered him the father of modern biochemical pharmacology (7, 10, 13).
Among his drug metabolism studies was reserpine, which had recently been introduced as a treatment for hypertension and schizophrenia. Reserpine has a very short half-life, but its pharmacological actions persisted long after the drug had been eliminated (13). Brodie wanted to know why.
Many researchers were speculating about reserpine’s effects in the brain (13). Brodie knew little about the brain, but his team noticed a structural similarity between reserpine and serotonin, and they had a tremendous advantage (7, 10, 11, 13). His colleagues, Sidney Udenfriend and Robert Bowman, had developed a new instrument, a spectrophotofluorometer, which could detect and measure serotonin and other biogenic amines in various tissues (7).
Using their new instrument, they showed that after administering reserpine to rats and rabbits, the content of serotonin in the animals’ brains became undetectable (11, 13). The results suggested to Brodie that depletion of brain serotonin was responsible for reserpine’s pharmacological effects. It was the first time an apparent link had been observed between brain biochemistry and important brain functions (7, 11).
In August 1955, a few months after Brodie’s reserpine discovery, Carlsson arrived. Brodie showed Carlsson his analytical methods and how to use his new spectrofluorometer (7, 10).
Carlsson proposed studying the effects of reserpine on catecholamines (i.e., epinephrine and norepinephrine), which are chemically similar to serotonin. Brodie thought that was a waste of time. He was convinced that serotonin was the key neurochemical (7, 10, 11). When Carlsson returned to Sweden in 1956, he still wanted to pursue catecholamines, but he needed a knowledgeable partner. Fortunately, Nils-Åke Hillarp, an expert in histology and histochemistry at the University of Lund, was interested. Hillarp also had extensive knowledge of physiology and biochemistry, which nicely dovetailed with Carlsson’s broad expertise in pharmacology (7, 10, 11).
In their first experiments, Carlsson and Hillarp found that reserpine depleted catecholamines from the adrenal medulla in rabbits (10). After purchasing a new commercially available Aminco-Bowman Spectrophotofluorometer, they demonstrated that reserpine also depleted catecholamines from the animals’ heart and brain (14). Because of reserpineinduced neurotransmitter depletion from nerve endings, sympathetic nerve stimulation no longer elicited a response. Carlsson concluded that depletion of catecholamines was also responsible for reserpine’s behavioral effects (7, 10, 11).
To test this, they gave DOPA to reserpine-pretreated animals. They chose DOPA, the biochemical precursor, because epinephrine and norepinephrine do not cross the blood-brain barrier (7, 10, 11). DOPA caused a dramatic reversal of the reserpine-induced behavioral syndrome in the animals (14). But to their surprise, DOPA administration did not restore the animals’ brain norepinephrine (14, 15).
To explain these effects, Carlsson was forced to look more closely at dopamine, the precursor, which until then had been disregarded because of its modest effect on smooth muscle preparations. No analytical method for differentiating dopamine from norepinephrine was available, so Carlsson and Hillarp had to develop one (10).
Using their new method in 1958, they showed that dopamine occurs normally in rabbit brains at slightly higher levels than norepinephrine, and dopamine disappears after reserpine treatment (15). DOPA restored brain dopamine levels, and that correlated with the ability of DOPA to reverse the behavioral syndrome induced by reserpine (15).
In addition to reserpine’s autonomic (cardiovascular) effects and calming effect in schizophrenia, clinicians were well aware that long-term reserpine treatment in
Early Stage Middle Stage Advanced Stage
Dopamine level
8 am Noon 4 pm 8 pm 8 am Noon 4 pm 8 pm 8 am Noon 4 pm 8 pm Natural dopamine level
Medicated dopamine level ↑ L-DOPA dose Therapeutic window
Off period Dyskinesia
L-DOPA is typically dosed 3 times daily. As Parkinson’s disease progresses, the therapeutic window narrows, natural dopamine levels decline, and higher and more frequent L-DOPA doses are required. Dyskinesias develop, typically corresponding to maximal L-DOPA levels in the brain. When L-DOPA levels drop below the therapeutic window, “off” period parkinsonian symptoms occur.
patients also caused a movement syndrome (rigidity, tremor, akinesia) indistinguishable from Parkinson’s disease (10, 16).
Carlsson’s observations led him to conclude that depletion of dopamine induces parkinsonism, and that treatment with L-DOPA would alleviate that syndrome by restoring brain dopamine levels (14). Carlsson presented all of this at the First International Catecholamine Symposium in October 1958 (7, 10).
These results did not attract much attention among neurophysiologists, but it was good enough for Carlsson to earn a promotion to Associate Professor at the University of Lund. In 1959 at the age of 36, he was appointed Professor and Chair of the Pharmacology Department at Göteborg University (11). Carlsson’s new job came with well-equipped research facilities (10).
Defying Dogma
In January 1959, Carlsson’s students, Åke Bertler and Evald Rosengren, found that the bulk of dopamine was in the striatum, the part of the brain known to control motor activity (8, 14, 17). They followed up by measuring dopamine in normal human brain specimens (8). In March 1960, Carlsson again presented his findings at the Symposium on Adrenergic Mechanisms in London. This meeting attracted the most eminent experts in the field, and they all held the view that the brain operated via electrical transmission (10).
No one questioned Carlsson’s results, but there was “profound and nearly unanimous skepticism” regarding dopamine’s role in the brain (10). Carlsson was still a relatively young and unestablished investigator, and he said, “the clear message to me was that I was a nobody!” (10).
Returning home from London, Carlsson and Hillarp resolved to intensify their efforts and “convince the world that chemical transmission does indeed exist in the brain” (10). They thought that the ability of catecholamines to fluoresce might allow them to visualize neuronal content of the compounds under the microscope (7, 10).
Hillarp developed a quantitative histofluorescent method (7, 11). Within a few years, Carlsson and Hillarp clearly established the neuronal localization of dopamine, norepinephrine, and serotonin (10). Others subsequently confirmed this and also found high dopamine levels in the substantia nigra and globus
pallidus (16). This led to mapping of the brain’s major monoaminergic pathways and provided irrefutable evidence to counter their critics (10, 11).
In February 1965, an international symposium, Mechanisms of Biogenic Amines, was held in Stockholm, again, with most of the leading experts in the field attending. This time, none of the participants doubted that amines play an important role in chemical neurotransmission in the brain (10).
It was a major turning point in neuroscience, not only acknowledging chemical transmission in the brain, but also inspiring investigators to determine the mechanisms of centrally acting drugs on brain amines (11). Carlsson said, he (like his mentor, Brodie) “simply had the advantage of being ignorant and not so much burdened by dogma” (10). Carlsson’s suggestion that lack of dopamine caused parkinsonian symptoms motivated clinical investigators on three continents to simultaneously and independently take the next logical step: conduct therapeutic clinical studies of Parkinson’s disease.
Japan
On August 5, 1959, Isamu Sano presented data showing that in the normal human brain, the bulk of dopamine was found in the striatum (8, 10, 17). It was the first clinical report of brain dopamine and further supported Carlsson’s view that dopamine might have a central role in the control of motor function (10, 17).
On February 6, 1960, Sano gave a lecture in Tokyo. He reported that in the post-mortem exam of a patient with Parkinson’s disease, he had found a low level of dopamine in the basal ganglia. Later that year, Sano also reported that intravenous administration of DOPA alleviated the rigidity of a patient with Parkinson’s disease (10).
Austria Clinical Proof
Like Arvid Carlsson, Oleh Hornykiewicz’s interest in dopamine began as a visiting researcher to a pharmacology lab. In 1956, Hornykiewicz went to Oxford University to work under Hermann Blaschko (8). Blaschko suspected that dopamine was biologically active, and he asked Hornykiewicz to pursue it. Using rabbits and guinea pigs, Hornykiewsicz confirmed Blaschko’s suspicion, showing that dopamine has physiological activity independent from norepinephrine and epinephrine (9).
After Hornykiewicz returned to Vienna, Austria, in February 1958, he saw several reports, including Carlsson’s 1958 paper reporting the presence of dopamine in rabbit brain. He adapted a colorimetric assay to measure dopamine and began his own studies of dopamine in rat brain homogenates (8).
Then, in 1959, Hornykiewicz saw Bertler and Rosengren’s and Sano’s publications. Along with his postdoctoral fellow, Herbert Ehringer, Hornykiewicz started collecting and analyzing human brains freshly obtained at autopsy. In April 1959, they obtained their first brain from a patient with Parkinson’s disease (8).
Hornykiewicz and Ehringer showed that the concentrations of dopamine, norepinephrine, and serotonin were markedly reduced in the caudate and putamen of patients with Parkinson’s disease, compared to control specimens (4, 5, 7, 17).
Their achievement was all the more remarkable, considering their austere facilities. They worked at a bench in a narrow semi-dark hallway in the basement of the pharmacology building. Without a spectrofluorometer, they relied on their comparatively crude colorimetric assay and a labor-intensive ionexchange chromatography separation (7, 8).
Interestingly, the color change was so dramatic that they could see the difference with the naked eye (8). In 1960, Hornykiewicz and Ehringer reported their findings, which, for the first time, linked neurochemical changes in the brain to the symptoms of Parkinson’s disease (5, 7, 8).
Those results provided a rationale for the next step: treating Parkinson’s disease with L-DOPA. Walther Birkmayer was caring for many patients with Parkinson’s disease at the Vienna Geriatric Hospital (8, 18). Hornykiewicz urged him to give those patients
L-DOPA (8). But Birkmayer had seen Brodie’s reports about reserpine-induced depletion of serotonin and clung to the idea that brain serotonin was responsible for parkinsonian symptoms (10, 18).
After nine months of Hornykiewicz’s “frequent reminders” and “pressure from above,” Birkmayer finally yielded (8, 18). Hornykiewicz gave Birkmayer his entire 2-gram supply of L-DOPA, which he had received from Hoffmann-LaRoche (18). At that time, it was a very precious compound, because of the difficulty and expense of producing the pure L-enantiomer. In July 1961, a skeptical Birkmayer gave 50 mg of L-DOPA intravenously to his first test patient. She improved so dramatically that it erased all his doubts (8, 18).
Birkmayer proceeded to treat 20 patients with up to 150 mg of L-DOPA. A home movie of some of the patients was shown at the Viennese Medical Society in November 1961. Hornykiewicz and Birkmayer’s published report gave an eloquent description of the drug’s effects:
“The effect of a single intravenous injection of L-DOPA was, in short, a complete abolition or substantial relief of akinesia. Bedridden patients who were unable to sit up, patients who could not stand up when seated, and patients who, when standing, could not start walking, performed all these activities with ease after L-DOPA. They walked around with normal associated movements, and they even could run and jump. The voiceless, aphonic speech, blurred by palilalia and unclear articulation, became forceful and clear as in a normal person” (8, 18).
Canada
André Barbeau spearheaded the parkinsonian research efforts in Canada. He conducted a great deal of experimental work on DOPA and the catecholamines. In patients with Parkinson’s disease, he found subnormal levels of dopamine excreted in the urine (5, 7).
Gerald Murphy (a graduate student at the time) and Ted Sourkes urged Barbeau to administer L-DOPA clinically. In 1962, they reported that L-DOPA exerted an anti-rigidity action in Parkinson’s disease, which appeared rapidly and lasted about 3 hours with a dose of 100 mg (5).
Convincing the Skeptics
Although the clinical results aroused great scientific interest, it took more than 5 years for L-DOPA to be implemented as a routine treatment for Parkinson’s disease (8, 10, 17). The initial treatment regimens provided only brief, marginal improvement, and as such, were thought to be of questionable clinical value (7).
George Cotzias, a senior scientist at Brookhaven National Laboratory and attending physician at Brookhaven National Laboratory Hospital, evaluated the early clinical reports and decided to administer higher doses, hoping to prolong DOPA’s effect (19).
He used the racemic D,L-DOPA, because the L-enantiomer was still very expensive (19). Sufficiently high oral doses of DOPA (3-16 grams per day) produced either a complete, sustained disappearance or a marked reduction of parkinsonian symptoms in 8 of his 16 patients. When the drug was discontinued, the full parkinsonian syndrome returned. Cotzias filmed some of his patients before and during therapy and published his results in 1967 (19).
Arvid Carlsson saw Cotzias’s convincing film at a meeting in Canada and hastened back to Göteborg, full of enthusiasm (10). He reached out to several Swedish clinicians, but they said replacement therapy for a neurodegenerative disease seemed “too fantastic to be seriously considered” (7).
Finally, one physician, Prof. Alvar Svanborg, agreed to conduct a clinical study. Then, Carlsson saw Svanborg’s patients, many of whom were in wheelchairs, and even he doubted that any drug could work. “But sure enough, in full agreement with Cotzias’s observations, a most dramatic result of this treatment soon became apparent” (7). Other clinical centers observed the same result, leading to widespread adoption of L-DOPA therapy (5).
Unfortunately, in addition to restoring dopamine levels in the brain, the high doses of L-DOPA also induced abnormally high levels of catecholamines in the rest of the body. And that caused an array of unpleasant side effects.
Carbidopa to the Rescue
Pharmacologists were initially interested in dopa decarboxylase (the enzyme that converts L-DOPA to dopamine) as a means of regulating norepinephrine at sympathetic nerve endings. Specifically, they thought that inhibiting dopa decarboxylase might lower blood pressure in hypertensive patients (5).
Researchers at the Merck Institute for Therapeutic Research synthesized many compounds, looking for a potent, selective inhibitor. Ultimately, they developed α-methyldopa, which was marketed to treat essential hypertension. Among the other inhibitors they synthesized was carbidopa (6).
Because carbidopa did not cross the blood-brain barrier, it inhibited dopa decarboxylase only in peripheral tissues, suppressing norepinephrine production in sympathetic nerve endings and reducing most of L-DOPA’s gastrointestinal and cardiovascular side effects. Co-administration of L-DOPA and carbidopa improved patient outcomes and is now the treatment of choice for Parkinson’s disease (5).
Mimicking Dopamine
Apomorphine, a natural product isolated from the roots and bulbs of water lilies, was used for decades as an emetic to treat poisoning (20). In the late 19th and early 20th centuries, it was also prescribed for sedation, analgesia, insomnia, schizophrenia, and erectile dysfunction (20, 21).
In 1951, investigators first administered sub-emetic doses of apomorphine to patients with Parkinson’s disease. The drug dampened rigidity and tremor. Even Cotzias continued to pursue apomorphine treatment after he demonstrated the efficacy of L-DOPA (5, 20). In the mid-1960s, researchers discovered that apomorphine is a dopamine agonist (5).
Nausea could be controlled with anti-emetic drugs, and apomorphine for Parkinson’s disease was introduced into clinical practice in the mid-1980s. Multiple clinical trials have since confirmed its efficacy, which equals L-DOPA. Due to extensive first-pass metabolism, apomorphine has a short oral half-life and must be given subcutaneously, which (along with emesis) limits its use (20).
In 1969, a 58-year-old woman who had contracted influenza was given the antiviral drug, amantadine (4, 5). The woman, who coincidentally suffered from Parkinson’s disease, experienced improvement in her parkinsonian symptoms. Later studies revealed that amantadine has mild dopamine effects (4, 5).
Other researchers focused on selective dopamine agonists, which they thought might avoid some of the side effects caused by L-DOPA (5). Experimental and clinical evidence indicates that activation of D2 receptors is beneficial in Parkinson’s disease, but dopamine agonists also stimulate other dopamine receptors to some extent (21).
By acting directly on dopamine receptors, dopamine agonists bypass the need for failing neurons to synthesize dopamine. Also, most dopamine agonists have much longer half-lives than L-DOPA, so they can produce a more sustained response (21).
The dopamine agonist, bromocriptine, is the prototype ergot derivative. In 1974, clinical trials showed that it was effective in treating Parkinson’s disease. All of the ergot alkaloids cause unpleasant side effects and liver and renal toxicity that limit their use (5).
The non-ergot dopamine agonists, such as pramipexole and ropinirole, were developed to provide therapeutic efficacy without the adverse effects of the ergot derivatives. In general, the clinical benefits of dopamine agonists are less than with L-DOPA, and they may be poorly tolerated by older patients (21).
Therapeutic Downside
Dopamine agonists are also associated with higher overall risk of adverse events than L-DOPA (3). The most critical of these side effects are fibrotic valvular heart disease and dopamine receptor-mediated impulsive/compulsive disorders (21).
Fibrotic valvular heart disease refers to functional abnormalities of the heart valve leaflets. It is
associated with long-term administration of the traditional ergot dopamine agonists (21). The risk of valve pathology may result from either high daily dosing or from cumulative dosing.
More than 40% of patients treated with oral dopamine agonists experience impulsive/compulsive disorders, especially at higher doses (3, 21). These non-motor effects (from excessive central dopaminergic stimulation) are a serious complication of long-term treatment.
The behaviors include gambling, compulsive spending, drug addiction, stereotyped behaviors such as inappropriate and unproductive fascination with common objects, pathological internet use, compulsive skin picking, binge eating, and hypersexuality (3, 21). Reducing the dopamine agonist dose and/or treatment with antidepressant drugs are effective remedies.
Unstoppable Degradation
The discovery of brain dopamine led to effective therapies, as James Parkinson anticipated, but not to a cure. Parkinson’s disease arises from degeneration of dopaminergic neurons in the nigrostriatal tract (5, 16, 21). Symptoms appear when 50-80% of those neurons are lost, and the severity of the disease correlates with the degree of loss (5, 21).
L-DOPA and the dopamine agonists aim to replace or substitute for neuronal dopamine. They improve motor (tremor, rigidity, akinesia) and nonmotor (constipation, cognition, mood, sleep) symptoms. But no existing drug treatment prevents neuronal loss or disease progression (3, 21).
Consequently, the clinical response to L-DOPA declines as the disease worsens (3, 17, 21). The therapeutic window narrows, requiring higher and more frequent drug doses. The resulting large swings in drug levels cause episodes of parkinsonian tremor and slowed movement when the drug level drops below the therapeutic window (“off” periods) and overdose side effects such as dyskinesia when the drug level peaks (3, 17).
The “off” periods can occur within 2 years of starting L-DOPA, and the frequency of “off” periods increases as the therapeutic window narrows (3).
Dyskinesias are involuntary twitching, jerky, or writhing movements of the face, limbs, or trunk. About 40% of patients experience dyskinesias after 4-6 years of L-DOPA treatment, and the risk of dyskinesia increases with higher L-DOPA doses (3). Dopamine agonists, which provide less robust symptom relief, have lower dyskinesia risk (3, 21).
Only apomorphine has efficacy comparable to L-DOPA, and it is an effective adjunct to oral dopaminergic treatment in advanced Parkinson’s disease. Injected apomorphine has a rapid onset and is effective in relieving “off” episodes, as well as suppressing dyskinesia (3, 20, 21). Co-administration with an antiemetic drug greatly increases tolerability.
In addition to severe “off” periods and dyskinesias, nonmotor symptoms increase as Parkinson’s disease progresses (3). Sensory symptoms include loss of smell, visual disturbances, and pain. Autonomic symptoms include constipation, orthostatic hypotension, and urinary dysfunction. Neuropsychiatric symptoms include anxiety, apathy, depression, and cognitive impairment (3).
Dopaminergic drugs generally provide little benefit, because many of these symptoms are mediated by non-dopaminergic pathways (3). Non-dopaminergic therapeutics include SSRIs for depression, antipsychotics for persistent psychosis, and cholinesterase inhibitors for cognitive impairment. Ultimately, most patients with Parkinson’s disease use drugs from multiple classes to attain complementary benefits while limiting high drug doses and doserelated adverse events (3).
Launching Neuroscience
The discovery, primarily by Arvid Carlsson, that dopamine is a neurotransmitter in the brain was a seminal event in the development of modern neuroscience (11, 17). He also showed that centrally acting drugs produced biochemical changes that correlated with changes in motor function. That insight led to L-DOPA therapy and development of more selective drugs for treating Parkinson’s disease.
In addition, researchers were inspired to conduct basic and clinical studies showing that centrally acting dopamine also mediates mood regulation, cognition, and reward. And dysfunction of those dopamine pathways is associated with major psychiatric diseases (8, 11, 17). But that’s another story.
For his many contributions to neuroscience, Arvid Carlsson received the Nobel Prize in 2000.
1. Bryson B (2003) A short history of nearly everything. Broadway Books, New York. 2. Parkinson J (1817) An essay on the shaking palsy, Sherwood, Neely, and Jones,
London. 3. Armstrong MJ and Okun MS (2020) Diagnosis and treatment of Parkinson Disease.
JAMA 323(6): 548-560. 4. Goetz CG (2011) The history of Parkinson’s Disease: Early clinical descriptions and neurological therapies. Cold Spring Harb Perspect Med 1:a0008862. 5. Sourkes TL and Gauthier S (1983) Levodopa and dopamine agonists in the treatment of Parkinson’s disease, In Discoveries in Pharmacology: Psycho- and
Neuro-pharmacology (Parnham MJ and Bruinvels J eds), pp 249-267, Elsevier, New
York. 6. Parascandola J (2010) Abel, Takamine, and the isolation of epinephrine. J Allergy
Clin Immunol 125(2): 514-517. 7. Carlsson A (2002) Treatment of Parkinson’s with L-DOPA. The early discovery phase, and a comment on current problems. J Neural Transm 109: 777-787. 8. Hornykiewicz O (2002) Dopamine miracle: From brain homogenate to dopamine replacement. Mov Disord 17(3): 501-508. 9. Barger G and Dale HH (1910) Chemical structure and sympathomimetic action of amines. J Physiol 41: 19-59. 10. Carlsson A (December 8, 2000) A half-century of neurotransmitter research: impact on neurology and psychiatry. Nobel Lecture; available from: https://www.nobelprize. org/uploads/2018/06/carlsson-lecture.pdf. 11. Rubin RP (2007) A brief history of great discoveries in pharmacology. Pharmacol
Rev 59(4): 289-359. 12. Arvid Carlsson – Biographical (May 6, 2022) NobelPrize.org. Nobel Prize Outreach
AB 2022; available from: https://www.nobelprize.org/prizes/medicine/2000/ carlsson/biographical/. 13. Costa E, Karczmar AG, and Vasell ES (1989) Bernard B. Brodie and the rise of chemical pharmacology. Annu Rev Pharmacol Toxicol 29: 1-21. 14. Carlsson A (1959) The occurrence, distribution and physiological role of catecholamines in the nervous system. Pharmacol Rev 11(2): 490-493. 15. Carlsson A, Lindqvist M, Magnusson T, and Waldeck B (1958) On the presence of 3-hydroxytyramine in brain. Science 127(3296): 471. 16. Hornykiewicz O (1966) Dopamine (3-hydroxytyramine) and brain function.
Pharmacol Rev 18(3): 925-964. 17. Björklund A and Dunnett SB (2007) Fifty years of dopamine research. Trends
Neurosci 30(5): 185-187. 18. Czech H and Zeidman LA (2014) Walther Birkmayer, co-describer of L-dopa, and his
Nazi connection: victim or perpetrator? J Hist Neurosci 23(2): 160-191. 19. Cotzias GC, Van Woert MH, and Schiffer LM (1967) Aromatic amino acids and modification of Parkinsonism. New Engl J Med 276(7): 374-379. 20. Carbone F, Djamshidian A, Seppi K, and Poewe W (2019) Apomorphine for
Parkinson’s disease: Efficacy and safety of current and new formulations. CNS
Drugs 33: 905-918. 21. Hisahara S and Shimohama S (June 13, 2011) Dopamine receptors and Parkinson’s disease. Int J Med Chem, Article ID 403039; doi:10.1155/2011/403039.
Biosketch:
Rebecca J. Anderson holds a bachelor’s in chemistry from Coe College and earned her doctorate in pharmacology from Georgetown University. She has 25 years of experience in pharmaceutical research and development and now works as a technical writer. Her most recent book is Nevirapine and the Quest to End Pediatric AIDS. Email rebeccanderson@msn.com.
In the next issue of The Pharmacologist…
Dr. Anderson will feature botox.
Don't miss the March 2023 issue.
Education News
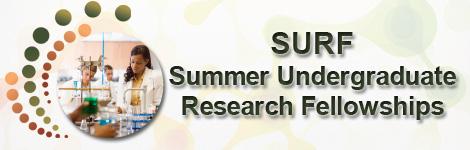
Individual Summer Undergraduate Research Fellowship (SURF) Program
Applications Due Tuesday, February 1, 2023, for Summer 2023 Fellowships
ASPET’s individual SURF program introduces undergraduate students to pharmacology research through a 10-week laboratory research experience. The goal of the program is to use authentic, mentored research experiences in pharmacology to heighten student interest in careers in research and related health care disciplines. The SURF individual awards are intended to support students whose institutions do not have a currently funded institutional SURF program. Research may be conducted at the student’s home institution or another institution, as appropriate to the research project.
Who Should Apply
Undergraduate students conducting pharmacologyrelated research including, but not limited to, students representing departments of pharmacology, toxicology, pharmaceutical sciences, and/or biological chemistry are invited to apply to the program. Applications from women and underrepresented minorities are particularly encouraged.
Program Details
■ Students must apply with a mentor who is a regular or affiliate member of ASPET in good standing or an emeritus member who is still active in research. ■ Students and mentors must have already identified, and briefly describe a summer research project that the student proposes to undertake. ■ If awarded, ASPET will provide a student stipend of $2800 for a minimum of ten weeks’ participation. ■ The student must apply for membership in AS-
PET no later than the beginning of their summer research experience.
For more information and to apply, please visit www. aspet.org/awards/SURF. For questions, please contact Catherine L. Fry, PhD at cfry@aspet.org.