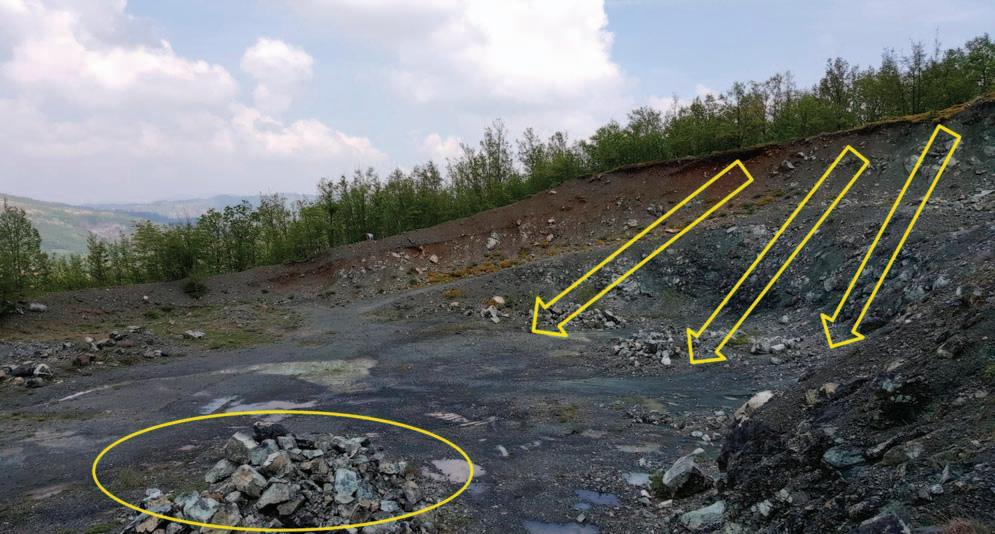
23 minute read
Naturally Occurring Asbestos: A Global Health Concern? State of the Art and Open Issues
from EEG Journal - February 2020 Vol. XXVI, No. I (2)
by Association of Environmental & Engineering Geologists (AEG)
ALESSANDRO F. GUALTIERI* Department of Chemical and Geological Sciences, University of Modena and Reggio Emilia, Via G. Campi 103, 41125, Modena, Italy
Key Terms: Asbestos, Environmental Geology, Hazardous Waste, Mining, NOA, Tunnels
ABSTRACT
Naturally occurring asbestos (NOA) is a global public health issue because minerals that may be classified as asbestos are a common constituent of certain types of rock and soil, found in many regions on every continent. Disturbance of these rocks and soils, especially through construction activities, can result in airborne particles, leading to inhalation and risk of disease from these known human carcinogens. The presence of NOA in the environment affects all the human activities aimed at its modification, and all engineering/geological actions in the natural environment should take it into account. In the presence of NOA, specific procedures for sampling, evaluation of environmental risk, and monitoring should be applied to minimize the risk of exposure for the workers and the general public. Unfortunately, detailed procedures have been lacking to date, and consensus is difficult to achieve because basic issues, such as the definition of asbestos itself, are still open and being debated by scientists and regulators. While the term “asbestos” has been used in older geological publications, it is not currently defined by geologists. For the past century, “asbestos” was a commercial term used to describe minerals mined for specific purposes, and the term then entered the legal lexicon for purposes of control and compensation. All these basic matters are critically illustrated in the article. Finding clear and universally accepted definitions is mandatory; otherwise, there will continue to be controversial positions that can cause regulatory and legal issues and the outcome of lawsuits to be very subjective.
INTRODUCTION
Naturally occurring asbestos (NOA) is a general term describing the six commercially classified forms of mineral fibers that are classified as asbestos when
*Corresponding author email: alessandro.gualtieri@unimore.it
found in their natural state (Lee et al., 2008). The definition of NOA pertains to the asbestos fibers that occur in rock and soil as result of natural geological processes (Harper, 2008) and not the asbestoscontaining manufactured products that can be eventually found in the environment. The term commonly applies to areas where asbestos minerals are found in such low quantities that mining and commercial exploitation are not viable. While large commercial deposits of asbestos minerals are relatively rare, small non-economic occurrences of asbestiform (defined as having at least one characteristic of asbestos, including being composed of bundles with frayed or splayed ends, being flexible, and having great tensile strength) minerals are more common (Lee et al., 2008). Strictly, the term NOA applies to the natural geologic occurrence of any of the six regulated types of asbestos minerals, so it should not include other mineral fibers, but it has been used in this more inclusive way. Natural weathering and/or human activities may disturb NOA-bearing rocks or soils and release asbestos into the air, inducing potential human exposure by inhalation.
Figure 1 shows a typical NOA site represented by an abandoned serpentine quarry in Romanoro, Northern Appennines (province of Modena, Italy). Although this quarry was not operated for asbestos production, asbestiform minerals have been identified in the rock. The rock piles and quarry faces from the former exploitation activity are persistently weathered by the action of atmospheric agents and percolating waters with a potential for the release of fibers to the air, although the extent of such release has not been quantified by air monitoring.
Anthropogenic activities, such as driving, cycling, hiking, and geological investigations in areas where asbestos minerals occur, can result in airborne fibers, although the exposure is likely to be very low unless the area is heavily contaminated as, for example, in the areas immediately adjacent to the old asbestos mines and tailings dumps in the Clear Creek Management Area of California.
NOA has raised concern worldwide with the appearance of scientific evidence of increased risk of malignant mesothelioma (MM) in the population
exposed to airborne asbestos released from NOA sites. An example is a California study where residential proximity to naturally occurring asbestos was associated with increased risk of MM (Pan et al., 2005), although the methodology in this study is easily criticized, for example, by noting that it took no account of the heavy rates of immigration and emigration in California.
The risk of MM decreased approximately 6.3% for every 10-km increase in residential distance from the nearest asbestos source (LaDou et al., 2010). However, studies of residents of Whatcom County, Washington, where gravels containing chrysotile have been extensively used by the community and where chrysotile is routinely deposited on residential property through river flooding, have shown no increase in morbidity despite the chrysotile presenting a much higher level of hazard than other types of asbestos in toxicological studies (Vander Kelen and Patrick, 2013). The greatest risk of exposure to asbestos-like minerals is in construction, and in some areas where these minerals are common, such as Fairfax County, Virginia, and in the state of California, specific procedures and regulations have been developed for construction activities (Lee et al., 2008).The presence of NOA in the environment needs to be considered in all the human activities aimed at its modification (e.g., engineering and geological activities, such as mining; tunnel, bridge, and dam construction; and road and highway pavement). In the presence of NOA, dedicated procedures for sampling, evaluation of environmental risk, and monitoring should be applied to reduce the risk of exposure for the workers and the general public. Regrettably,
Figure 1. A typical example of NOA site represented by an abandoned serpentine quarry, specifically the serpentine rock quarry of Romanoro in the northern Appennines (province of Modena, Italy). Rock piles and quarry faces from the former exploitation activity are persistently leached by the action of atmospheric agents (namely, wind and rain) and percolating waters, releasing chrysotile- and tremolite-rich airborne particulate. Anthropogenic actions may also cause disturbance (original picture A. F. Gualtieri).
shared unambiguous procedures are lacking to date because there is no international platform for addressing this issue. Limits of the existing definitions include (1) the mineral fibers to be classified and regulated as asbestos, (2) correct measurement of mineral fibers to be properly classified as asbestos, (3) the determination of the concentration of asbestos and mineral fibers in massive materials, (4) if elongate mineral particles other than those already classified as asbestos represent a hazard, and (5) the toxicity/pathogenicity potential of mineral fibers other than those classified as asbestos.
As discussed in the core of this article, if we do not attempt to solve these basic issues, there will always be controversial positions, legal issues, and subjective outcomes of worldwide lawsuits, ultimately delaying the protections necessary to preserve human health.
THE PROBLEM OF THE DEFINITION OF ASBESTOS
As remarked by Harper (2008), the risk to health when living on soil and rock in the presence of NOA is not so obvious. The picture becomes even less clear when the minerals are subject to intensive investigation since our generally accepted definitions of asbestos are themselves put to the test. The discovery of asbestos or related minerals has consequences beyond any immediate risks to health, including profound effects on the value of and ability to use or enjoy property. To date, the literature reports at least 15 different definitions of asbestos (Gualtieri, 2017), and despite the fact that classification of asbestos minerals has been studied for about 40 years, there is still controversy as to which mineral particles should be classified as asbestos (Gualtieri et al., 2018b). Regrettably, the considerations made to date do not correspond to the common understanding of what asbestos is, and, to some degree, these two approaches sometimes contradict one another (Case et al., 2011). One of the early regulatory definitions of asbestos is the following: “Six fibrous silicates are classified as ‘asbestos’: the fibrous serpentine called chrysotile and the fibrous amphiboles actinolite, amosite, anthophyllite, crocidolite and tremolite” (U.S. Department of Labor, 1975). Since the 1970s, many other definitions have been proposed, but invariably these were ambiguous or had contradictory aspects. The National Institute for Occupational Safety and Health (NIOSH, 2011) has reviewed numerous resources and has not found any current reference for standard terminology and definitions that is complete and unambiguous. For example, in 1980, NIOSH and the Occupational Safety and Health Administration (OSHA) used the following definition for
workplace exposure to airborne asbestos in the United States (review and recommendations 81,103): “1. Asbestos is defined to be chrysotile, crocidolite, and fibrous cummingtonite-grunerite including amosite, fibrous tremolite, fibrous actinolite, and fibrous anthophyllite. 2. The ‘fibrosity’ of the above minerals is ascertained on a microscopic level with fibers defined to be particles with an aspect ratio 3:1.” While this definition may be appropriate for airborne asbestos particles, it is hardly applicable to bulk rocks and minerals since asbestos was prized and mined not for being composed of microscopic fibers but rather for fibers that were centimeters or even meters long that, for example, could be woven into cloth. The major stumbling block in the appreciation of asbestos has been the substantial disconnect between the macroscopic material that occurs in the earth, which was mined and used commercially, and the microscopic materials generated from it, which can lead to disease. This definition is a first attempt to give a definition of “microscopic fiber” but is too generic. There are no constraints on the length and width (or diameter) of the particles except that length and width are practically constrained by the methodology of sampling airborne particulates, which does not collect particles above a certain length or width. Particles with an aspect ratio (length/width, by geometric definition) >3 can display a non-fibrous habit (bladed, lamellar, columnar, or prismatic), but the definition is to be applied in asbestos mines and product factories, where the vast majority of particles with an aspect ratio >3 will be asbestos. Nevertheless, the OSHA analytical method includes procedures (albeit subjective) for the exclusion of non-fibrous particles, as these are not included in OSHA regulations.
The definition of asbestos has been strongly linked to the concept of “fiber” on a microscopic level. Inadequate and incomplete definitions of asbestos have resulted, as noted by an International Agency for Research on Cancer (IARC) consensus panel, in “taxonomic confusion and lack of standardized operating definitions for fibers.” The term “asbestos” is often inappropriately used as a generic, homogeneous rubric, and even when an asbestos fiber type is specified, its source is rarely stated (Kane et al., 1996).
Ambiguity in the definition of asbestos may lead to widespread confusion (Lee et al., 2008) and difficulties in properly handling activities in the presence of NOA. It is likely that no scientist would disagree that if we could turn back time and eliminate the word “asbestos,” we would. “Asbestos” has been used, without a good definition and not always in the same way, in commerce, regulation and law, and the health-related professions to such an extent that we are “stuck with it.” It is probably not now definable in any scientifically defensible way, and any further attempts to refine such a definition would then be inevitably doomed.
THE PROBLEM OF THE DEFINITION OF “ASBESTOS FIBER”
Any definition of “asbestos” could not be separated from a definition of “fiber.” Being fibrous or not makes a difference (e.g., chrysotile, the fibrous variety of serpentine, is classified as asbestos, whereas lizardite, the lamellar variety of serpentine, is not classified as asbestos). Unfortunately, as with asbestos, there is no clear agreement on what we call a “fiber” (Belluso et al., 2017) other than it must be an object with elongation in one dimension compared to the other two. Although pictures of objects have been drawn in an attempt to guide others in the selection of terms such as “fibrous,” “acicular,” or “prismatic,” it remains that none of these terms has a good consensus definition.
Because fiber is the issue of concern for the healthrelated professions (medical, epidemiological, and toxicological) and thus, in turn, for the regulatory and legal professions, a definition could be considered critical (Belluso et al., 2017). One may be tempted to use the World Health Organization (1997) guidelines for the determination of airborne fiber number concentrations: “Airborne fibers are objects with a length L >5µm, a width W <3µm, and L:W (aspect ratio) >3:1, using a phase-contrast optical microscope (PCOM).” However, this is not a definition but simply counting criteria, a protocol to count inhalable particles able to penetrate down to the alveolar space using PCOM. It is considered an index of exposure to airborne asbestos particles, and the value lies in its traceability to a body of measurements that could be compared to the prevalence of disease in workers whose exposures were measured in accordance with this procedure, thus allowing a dose–response relationship for use in risk assessment. Such parameters come from the assumption that the fiber length (L), width (W), and biopersistence (B) are the bearing walls of the fiber toxicity paradigm: long, thin and biopersistent fibers have the highest potential to induce adverse effects in vivo (Figure 2) and prompt carcinogenesis. However, others have proposed different dimensional definitions (e.g., >20:1; Chatfield, 2008). Even in regulations, we find differences. For example, >3:1 is considered the proper aspect ratio definition in some areas of jurisdiction but >5:1 in others, and while the majority count particles longer than 5 µm, others use 0.5 µm. Different definitions are sometimes for different purposes, for example, one definition to determine presence but another to determine risk.
The issue is further confounded when attempting to apply definitions of fiber to bulk materials and their
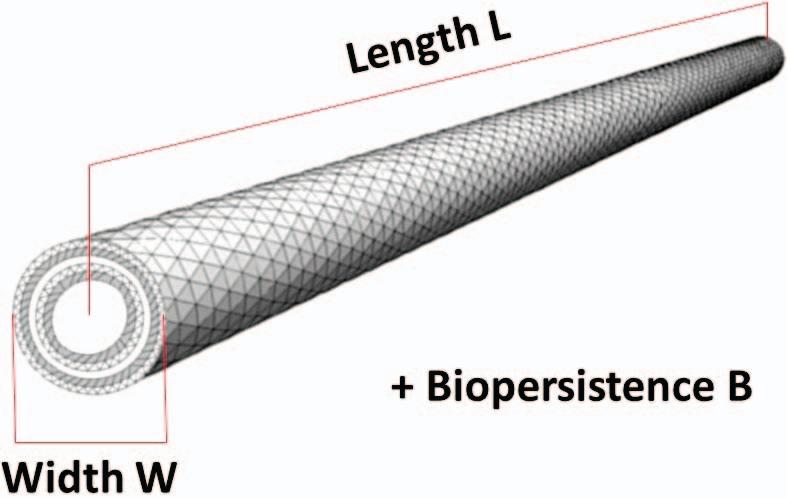
Figure 2. The three parameters defining the fiber toxicity paradigm: fiber length (L), fiber width (W), and biopersistence (B).
likely ability to release microscopic fibers. Microscopic fibers may be revealed by direct investigation of macroscopic materials, but far more microscopic fibers may be released by the handling of these materials, and the degree to which they will be released is a function of the type of handling and the energy involved. No proposals for a standardized procedure to determine the propensity of a bulk material to release microscopic fibers to the air have been agreed. Thus, it remains impossible to compare across studies and properly evaluate the relative risk of materials and processes.
THE PROBLEM OF THE MEASUREMENT OF THE AIRBORNE CONCENTRATION OF ASBESTOS
Because no unequivocal definition of asbestos exists, no unequivocal analytical procedures for identification and measurement of airborne concentration exist. All the available methods are the result of approximations and compromises and are invariably affected by some degree of bias and uncertainty. As noted above, the procedures using optical microscopy were developed for use in the asbestos industry (and were later expanded to include remediation of asbestos in buildings and polluted areas) but were never designed for the measurement of asbestos in ambient air, where there may be substantial presence of non-asbestos particles, both mineral and of biological origin, to interfere with the observation. Methods involving transmission electron microscopy and scanning electron microscopy have been developed to improve the situation, but these methods lack the underpinning of a dose–response relationship where dose has been assessed from either technique, and thus such measurements cannot be directly applied to risk assessment. Since such a relationship has been determined for the optical microscopy method, some measure of equivalence to the optical microscopy measurements is assumed for the purpose. When these methods developed to the determination of airborne asbestos fibers are applied to the study of massive matrices, the situation is even more challenging. An open issue is the definition of the detection limits. For the Italian law (D.M. 471/1999), the asbestos limit concentration must not exceed 1 g of fibers for every kilogram of dry soil (0.1 wt%). However, the accurate and precise quantification of asbestos at or below 1 wt% in a real ground sample is an open analytical challenge (Foresti et al., 2003). Furthermore, the analysis of NOA in massive samples is complicated by the fact that the host matrix often shares the very same elemental composition and/or crystallographic features with asbestos. Electron microscopies, capable of discriminating the non-fibrous matrix from asbestos in natural samples, are preferred experimental methods with transmission electron microscopy that offers the highest resolution and the most accurate fiber identification but requires long analysis time for a statistically relevant quantitative analysis (Cossio et al., 2018). New analytical procedures appear in the literature to overcome the intrinsic limitations of these experimental methods. An example is described in Cossio et al. (2018), who developed a fully automatic quantification of asbestos fibers by scanning electron microscopy and energy dispersive X-ray spectroscopy to quantify asbestos in complex and confounding matrixes, including amphibole- and serpentine-rich ophiolites.
THE PROBLEM OF THE ELONGATE MINERAL PARTICLE
Because of the complicated issue of the definition and measurement of “asbestos,” “asbestiform,” “fiber,” and related terms, some years ago, a new term, elongate mineral particle (EMP), was coined for use in the NIOSH (2011) “roadmap” for the purpose of classifying all fiber-like mineral particles under a single term. Gunter (2009) noted issues around the use of the term “NOA,” where a constant component of the rocks and soils at NOA sites are elongate mineral particles unlikely to be classified as asbestos together with elongate mineral particles that would likely be classified as asbestos.
By definition, any mineral particle with a minimum aspect ratio of 3:1 is considered an EMP (Belluso et al., 2017). A special case of EMP is the “elongate cleavage fragment” (ECF): “any particle with the same chemical formula of the asbestiform variety is considered an ECF if it is originated from a crystal that cleaves into fragments rather than separates longitudinally into fibrils” (Gunter et al., 2007). It should be remarked that there is widespread distribution of amphiboles in the
natural environment that can also occur with a 3:1 aspect ratio or greater when crushed. Thus, if the term EMP is to be used, it will require a more thorough description than a 3:1 aspect ratio, as this will make most natural mineral-containing materials (i.e., soils and rocks) “contaminated” with EMPs (Gunter, 2018). There are then two issues regarding ECFs. First, they must be broken from the original particle, and this is less likely to have occurred in situ, so that they will be more likely present in aerosol derived from disturbing the bulk than in the bulk. Second, to be separated for the purpose of risk assessment, they should present little or no hazard. While OSHA and some other national regulatory bodies do not regulate cleavage fragments, both NIOSH and the Environmental Protection Agency found insufficient evidence for a sufficiently low risk of disease, so that inclusion with asbestos is their current position of prudence.
The discussion at the recent Monticello Conference on EMP, held in Charlottesville, Virginia, in 2017 (Weill, 2018), pointed out that lack of precision with terminology and clear definition of EMPs have impeded the scientific community’s ability to properly characterize health effects attributable to specific types of EMPs. There was broad agreement with the original usage by NIOSH that the term “EMP” does not speak specifically about the human health effects of the substances categorized under this umbrella term (Weill, 2018). Moreover, it was highlighted that the utilization of the aspect ratio alone should be avoided in EMP characterization and, again, that the term EMP does not alone imply elevated risk for an asbestos-related disease. A good example of this is the zeolite erionite. While exposure to airborne erionite is associated with MM, especially within a specific Turkish population, the same exposures, unlike with asbestos, have not also resulted in elevated rates of lung cancer. However, such differences also occur in minerals traditionally classified as asbestos; the MM rate for workers exposed only to chrysotile, a serpentine mineral, is undeniably much lower than for cohorts exposed to chrysotile and amphibole minerals or to amphiboles alone. The rates are in fact so low that some researchers were led to declare that MM resulted from only amphibole exposure (the “amphibole hypothesis”). Hence, while there may be a belief in some quarters that EMP is an overly inclusive term, the same is also true for asbestos.
THE PROBLEM OF THE NATURALLY OCCURRING MINERAL FIBERS (NOMF) OTHER THAN ASBESTOS
There are at least 400 known mineral species that display a fibrous (or fibrous-asbestiform) crystal habit. Besides the six asbestos minerals, there are few regulated fibers classified as carcinogenic by the IARC, namely, erionite and fluoro-edenite. In those cases, the general term NOA is no longer correct and should be modified according to the specific case (e.g., NOE for naturally occurring erionite). The rest of the mineral fibers are not regulated or classified in terms of potential toxicity/pathogenicity (Gualtieri 2017, 2018). There are many examples of natural occurrence of unclassified mineral fibers that may represent a potential hazard in Europe and the United States. Potential public exposure to respirable mineral dust other than asbestos from natural deposits is an issue of great concern in the United States (Swayze et al., 2009; California Air Resource Board, 2017). In California, NOMF (mostly NOA) occurs in 90% of the 58 counties. A remarkable example of unregulated potentially toxic/pathogenic fiber found in the blueschists of the Franciscan Complex (California) is fibrous glaucophane (Erskine and Bailey, 2018). The Franciscan Complex rocks are commonly excavated locally for building/construction purposes in northern and central California (e.g., the Calaveras Dam Replacement Project as well as many developmental projects in the city and county of San Francisco), and the dust generated by the excavation activities may potentially expose construction workers and the nearby populations to adverse health risks.
Another example is represented by the fibrous zeolite ferrierite that occurs in southern Nevada. A recent study showed that fibrous ferrierite has the same chemical-physical properties that are deemed to prompt adverse effects in vivo by erionite (Gualtieri et al., 2018a). However, no restriction has been considered against it because neither toxicity/carcinogenicity nor epidemiological studies have been conducted to date.
CONCLUSIONS
This article describes the major problems encountered by the engineering/geological community when facing activities in the presence of NOA. Major problems are related to the lack of unique definitions and procedures for the identification and analysis of asbestos-containing materials worldwide. When dealing with asbestos-related problems, ambiguity in the definitions, health effects of asbestos and non-asbestos species, and experimental methods may lead to widespread confusion and miscommunication, which in turn can lead to unnecessary media hysteria and public alarm (Lee et al., 2008). Ambiguity in the regulatory definitions may also prompt twisted science and legal disputes.
In perspective, the global academic and engineering/geological community should work in synergy by
sharing experiences and overcoming ambiguities to achieve common and safe procedures to work in the presence of NOA. Specific solutions to problems such as mitigation of NOA during excavation, tunneling, and mining activity are necessary. To this aim, the continued efforts of dedicated working groups and commissions is highly recommended.
ACKNOWLEDGMENTS
The author wishes to thank the three competent referees for the work and especially Dr. M. Harper, who was of great help in improving the quality of the final version of this article.
REFERENCES
Belluso, E.; Cavallo, A.; and Halterman, D., 2017, Crystal habit of mineral fibres. In Mineral Fibres: Crystal Chemistry, Chemical-Physical Properties, Biological Interaction and Toxicity European Mineralogical Union-EMU Notes in Mineralogy, Vol. 18, pp. 65–109. California Air Resource Board, 2017, Implementation Guidance Document, Air Resource Board Test Method. Determination of Asbestos Content of Serpentine Aggregate: Field and Laboratory Practices ARB Monitoring and Laboratory Division, Quality Management Branch, Quality Management Section. 435 p. Case, B. W.; Abraham, J. L.; Meeker, G. D. P. F.; Pooley, F. D.; and Pinkerton, K. E., 2011, Applying definitions of “asbestos” to environmental and “low-dose” exposure levels and health effects, particularly malignant mesothelioma: Journal of Toxicology and Environmental Health, Part B, Vol. 14, No. 1–4, pp. 3–39. Chatfield, E. J., 2008, A procedure for quantitative description of fibrosity in amphibole minerals. In 2008 Johnson Conference: Critical Issues in Monitoring Asbestos: ASTM International, Burlington, Vermont. pp. 53–54. Cossio, R.; Albonico, C.; Zanella, A.; Fraterrigo-Garofalo, S.; Avataneo, C.; Compagnoni, R.; and Turci, F., 2018, Innovative unattended SEM-EDS analysis for asbestos fiber quantification: Talanta, Vol. 190, pp. 158–166. Erskine, B. G. and Bailey, M., 2018, Characterization of asbestiform glaucophane-winchite in the Franciscan Complex blueschist, northern Diablo Range, California: Toxicology and Applied Pharmacology, Vol. 361, pp. 3–13. Foresti, E.; Gazzano, M.; Gualtieri, A. F.; Lesci, I. G.; Lunelli, B.; Pecchini, G.; Renna, E.; and Roveri, N., 2003, Determination of low levels of free fibres of chrysotile in contaminated soils by X-ray diffraction and FTIR spectroscopy: Analytical and Bioanalytical Chemistry, Vol. 376, No. 5, pp. 653–658. Gualtieri, A. F., 2017, Mineral Fibres: Crystal Chemistry, Chemical-Physical Properties, Biological Interaction and Toxicity. EMU Notes in Mineralogy 18: European Mineralogical Union, Jena, Germany. 536 p. Gualtieri, A. F., 2018, Towards a quantitative model to predict the toxicity/pathogenicity potential of mineral fibers: Toxicology and Applied Pharmacology, Vol. 361, pp. 89–98. Gualtieri, A. F.; Gandolfi, N. B.; Passaglia, E.; Pollastri, S.; Mattioli, M.; Giordani, M.; Ottaviani, M. F.; Cangiotti, M.; Bloise, A.; Barca, D.; Vigliaturo, R.; Viani, A.; Pasquali, L.; and Lassinantti Gualtieri, M., 2018a, Is fibrous ferrierite a potential health hazard? Characterization and comparison with fibrous erionite: American Mineralogist, Vol. 103, No. 7, pp. 1044–1055. Gualtieri, A. F.; Gandolfi, N. B.; Pollastri, S.; Rinaldi, R.; Sala, O.; Martinelli, G.; Bacci, T.; Paoli, F.; Viani, A.; and Vigliaturo, R., 2018b, Assessment of the potential hazard represented by natural raw materials containing mineral fibres—The case of the feldspar from Orani, Sardinia (Italy): Journal of Hazardous Materials, Vol. 350, 76–87. Gunter, M. E.; Belluso, E.; and Mottana, A., 2007, Amphiboles: Environmental and health concerns: Reviews in Mineralogy and Geochemistry, Vol. 67, No. 1, pp. 453–516. Gunter, M., 2009, Asbestos sans mineralogy: Elements, Vol. 5, No. 3, p. 141. Gunter, M. E., 2018, Elongate mineral particles in the natural environment: Toxicology and Applied Pharmacology, Vol. 361, pp. 157–164. Harper, M., 2008, 10th anniversary critical review: Naturally occurring asbestos: Journal of Environmental Monitoring, Vol. 10, No. 12, pp. 1394–1408. Kane, A. B.; Boffetta, P.; Saracci, R.; and Wilbourn, J. D., 1996, Mechanisms of Fibre Carcinogenesis: International Agency for Research on Cancer Scientific Publication 140. LaDou, J.; Castleman, B.; Frank, A.; Gochfeld, M.; Greenberg, M.; Huff, T. K. J.; Landrigan, P. J.; Lemen, R.; Myers, J.; Soffritti, M.; Soskolne, C. L.; Takahashi, K.; Teitelbaum, D.; Terracini, B.; and Watterson, A., 2010, The case for a global ban on asbestos: Environmental Health Perspectives, Vol. 118, No. 7, pp. 897–901. Lee, R. J.; Strohmeier, B. R.; Bunker, K. L.; and Van Orden, D. R., 2008, Naturally occurring asbestos—A recurring public policy challenge: Journal of Hazardous Materials, Vol. 153, No. 1–2, pp. 1–21. National Institute for Occupational Safety and Health, 2011, Asbestos Fibers and Other Elongate Mineral Particles: State of the Science and Roadmap for Research: Department of Health and Human Services, Centers for Disease Control and Prevention National Institute for Occupational Safety and Health. 147 p. Pan, X. L.; Day, H. W.; Wang, W.; Beckett, L. A.; and Schenker, M. B., 2005, Residential proximity to naturally occurring asbestos and mesothelioma risk in California: American Journal of Respiratory and Critical Care Medicine, Vol. 172, No. 8, pp. 1019–1025. Swayze, G. A.; Kokaly, R. F.; Higgins, C. T.; Clinkenbeard, J. P.; Clark, R. N.; Lowers, H. A.; and Sutley, S. J., 2009, Mapping potentially asbestos-bearing rocks using imaging spectroscopy: Geology, Vol. 37, No. 8, pp. 763–766. U.S. Department of Labor, 1975, Occupational exposure to asbestos: Federal Regulation 40, pp. 47652–47665. Vander Kelen, P. and Patrick, G., 2013, Sumas Mountain/Swift Creek Asbestos Cluster Investigation: Electronic document, available at http://www.doh.wa.gov/Portals/1/ Documents/Pubs/334-331.pdf. Weill, D., 2018, Proceedings of the Monticello Conference on Elongate Mineral Particles (EMP): Toxicology and Applied Pharmacology, Vol. 361, pp. 1–2. World Health Organization, 1997, Determination of Airborne Fibre Number Concentrations: World Health Organization, Geneva, Switzerland.