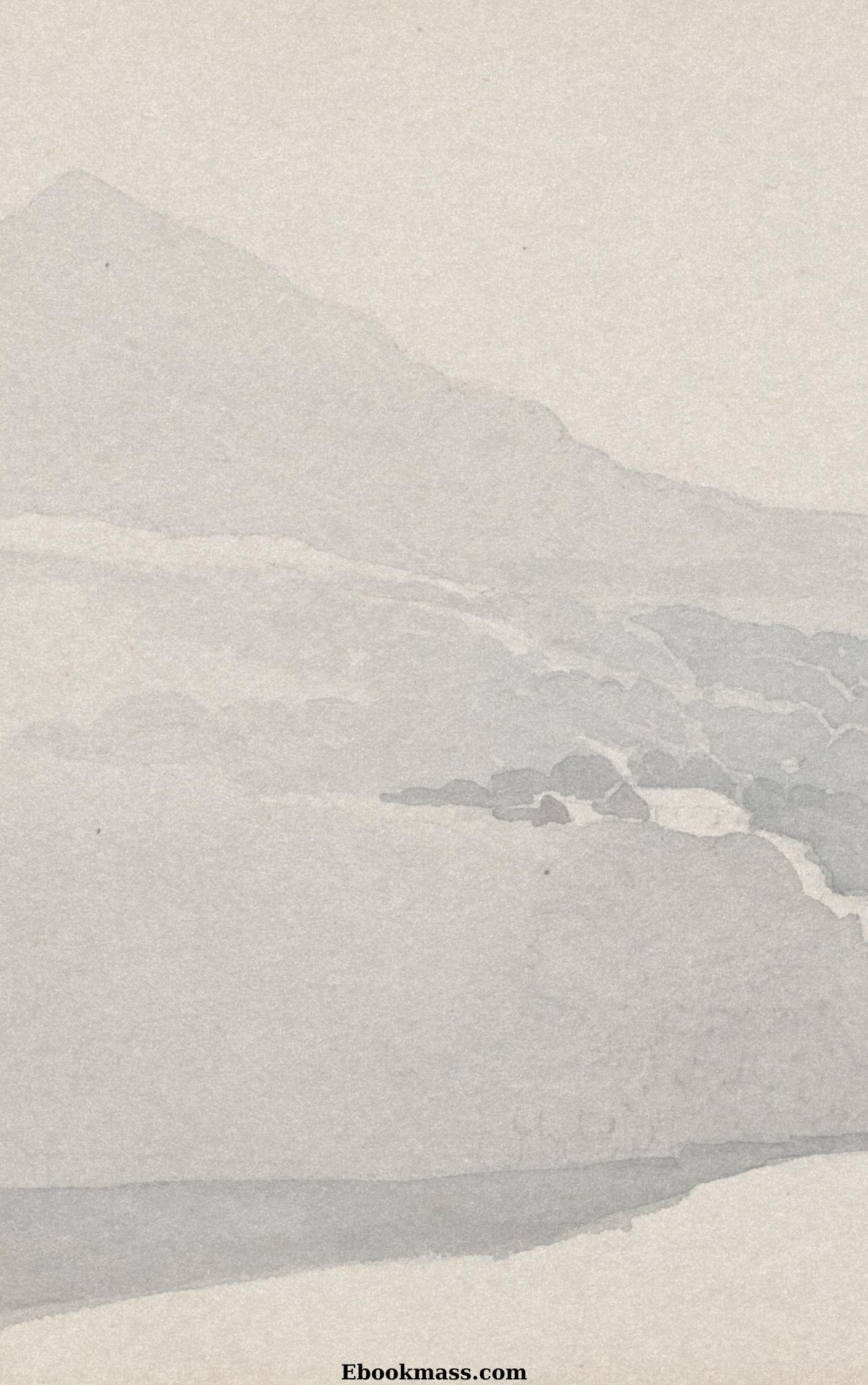
Improvedmethanolelectrooxidationcatalyzedby orderedmesoporousPt-Ru-Iralloynanostructures withtraceIrcontentSabarinathanRavichandran& NarayanamoorthyBhuvanendran&QianXu& ThandavarayanMaiyalagan&HuanengSu https://ebookmass.com/product/improved-methanolelectrooxidation-catalyzed-by-ordered-mesoporous-pt-ru-iralloy-nanostructures-with-trace-ir-content-sabarinathanravichandran-narayanamoorthy-bhuvanendran-qian-xuthandavaraya/
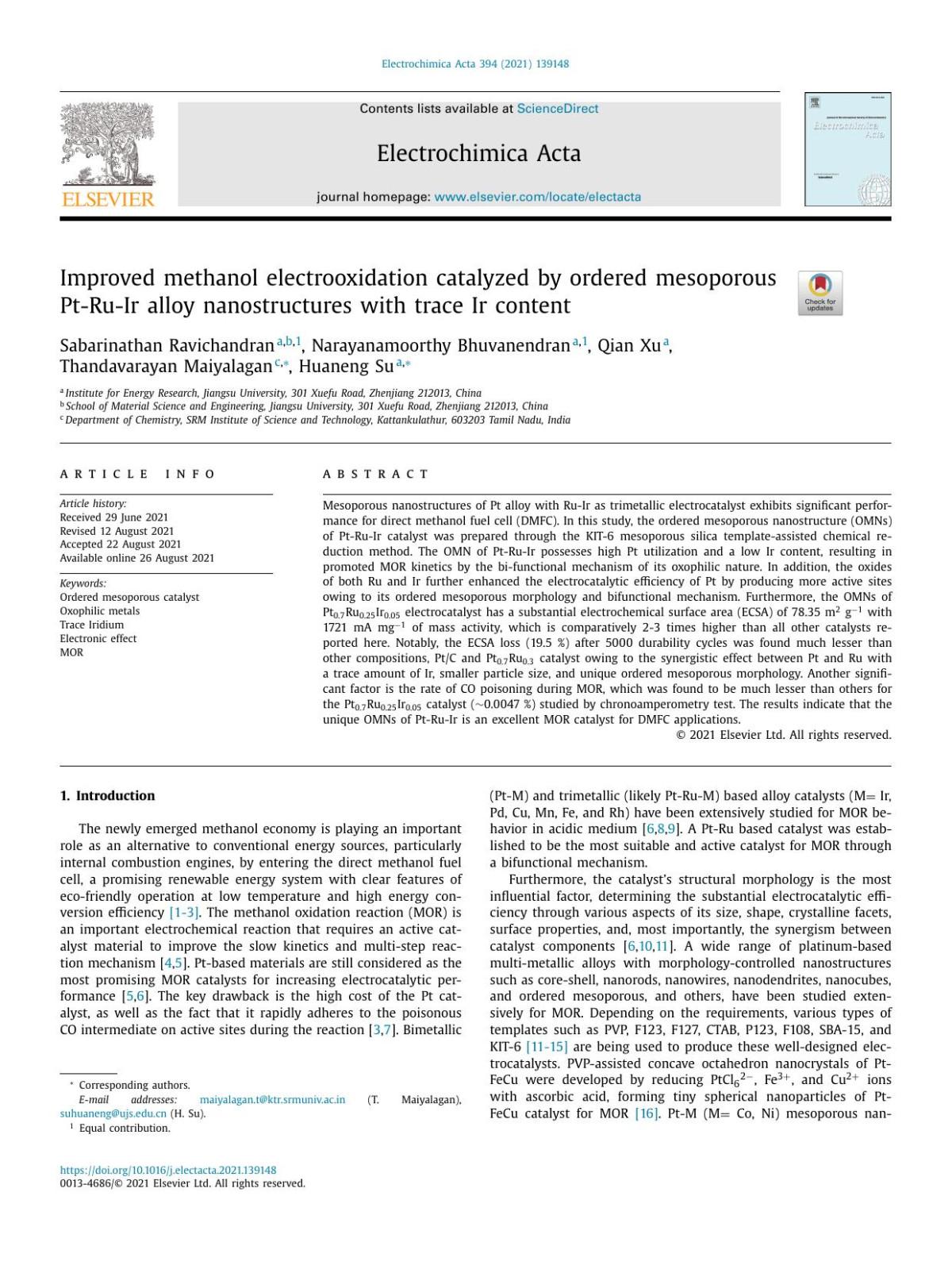
Instant digital products (PDF, ePub, MOBI) ready for you
Download now and discover formats that fit your needs...
Pt-based (Zn, Cu) nanodendrites with enhanced catalytic efficiency and durability toward methanol electrooxidation via trace Ir-doping engineering Kai Peng & Weiqi Zhang & Narayanamoorthy Bhuvanendran & Qiang Ma & Qian Xu & Lei Xing & Lindiwe Khotseng & Huaneng Su
https://ebookmass.com/product/pt-based-zn-cu-nanodendrites-withenhanced-catalytic-efficiency-and-durability-toward-methanol-electrooxidation-via-trace-ir-doping-engineering-kai-peng-weiqi-zhangnarayanamoorthy-bhuvanendra/ ebookmass.com
Elsevier Weekblad - Week 26 - 2022 Gebruiker
https://ebookmass.com/product/elsevier-weekbladweek-26-2022-gebruiker/
ebookmass.com
Jock Seeks Geek: The Holidates Series Book #26 Jill Brashear
https://ebookmass.com/product/jock-seeks-geek-the-holidates-seriesbook-26-jill-brashear/ ebookmass.com
The Essential Entrepreneur: What It Takes To Start, Scale And Sell A Successful Business 1st Edition Richard Turner
https://ebookmass.com/product/the-essential-entrepreneur-what-ittakes-to-start-scale-and-sell-a-successful-business-1st-editionrichard-turner/ ebookmass.com
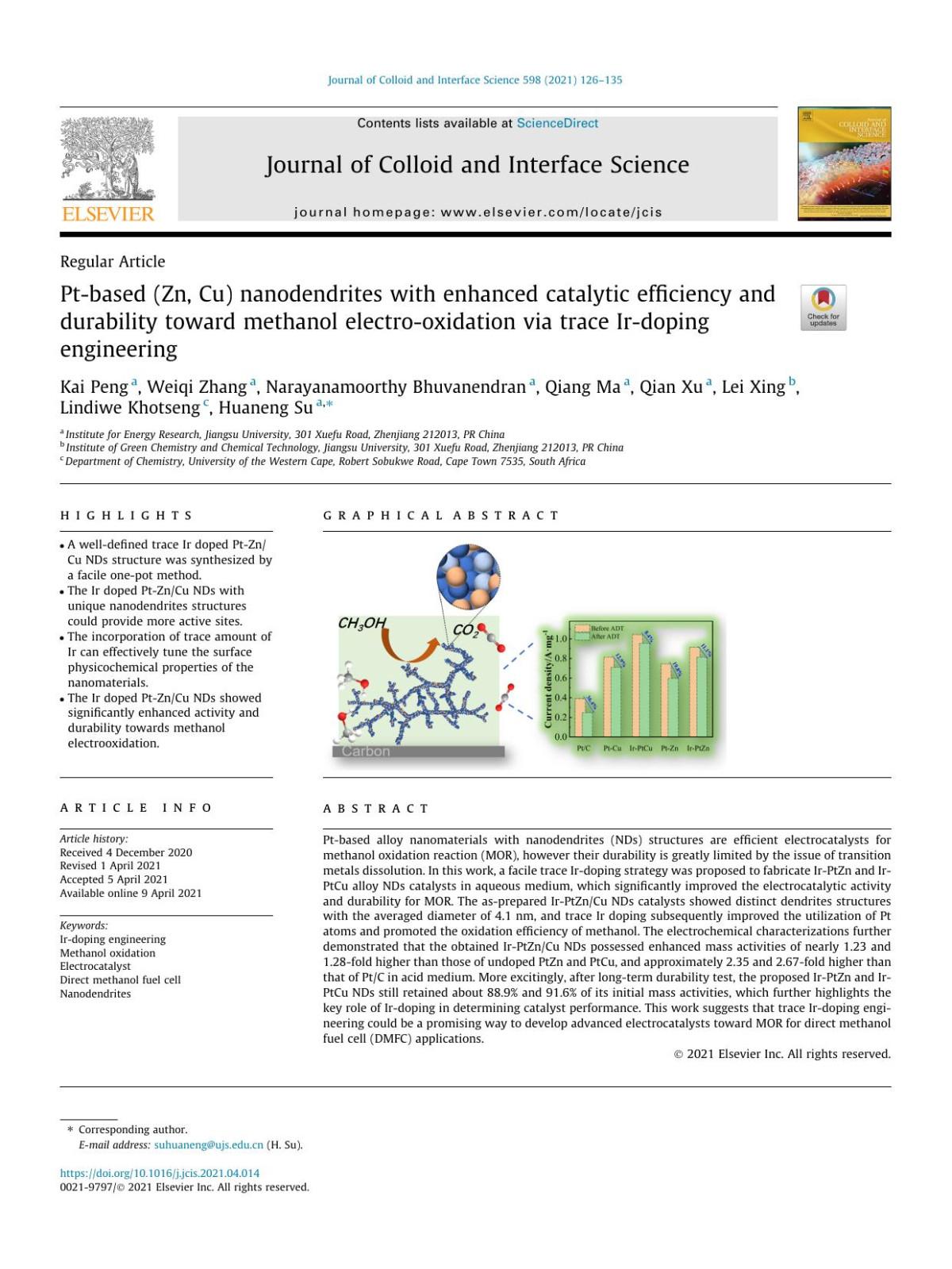
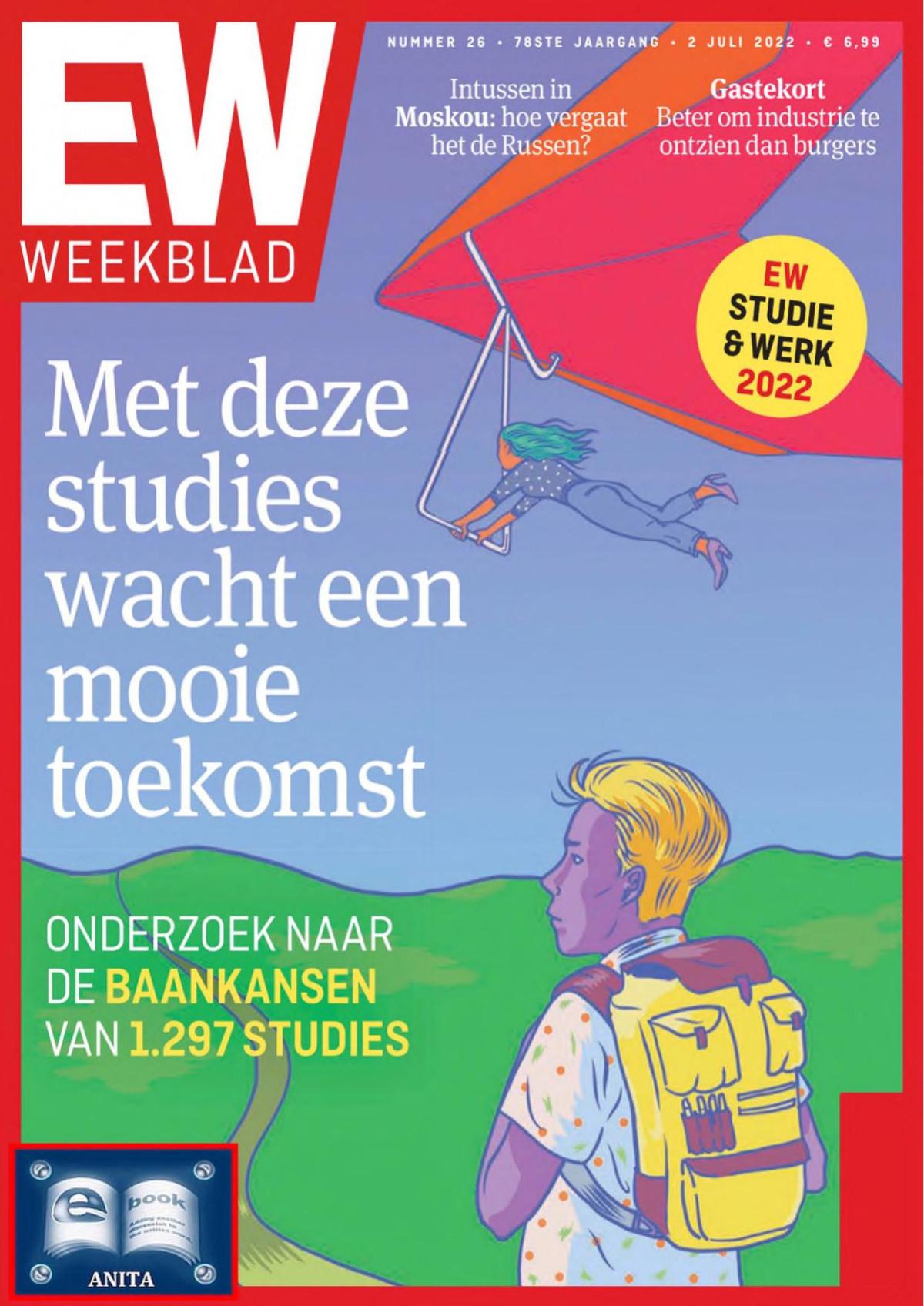
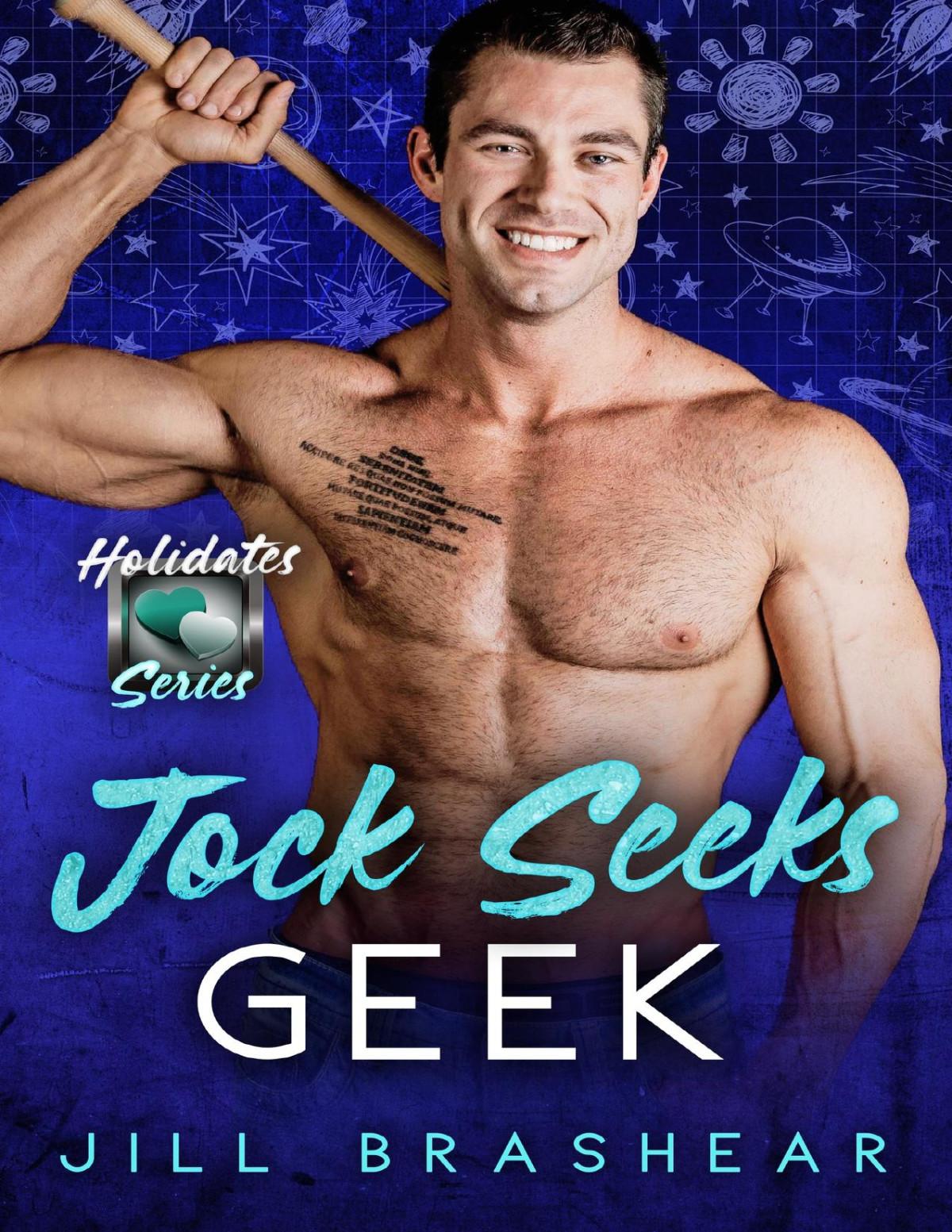
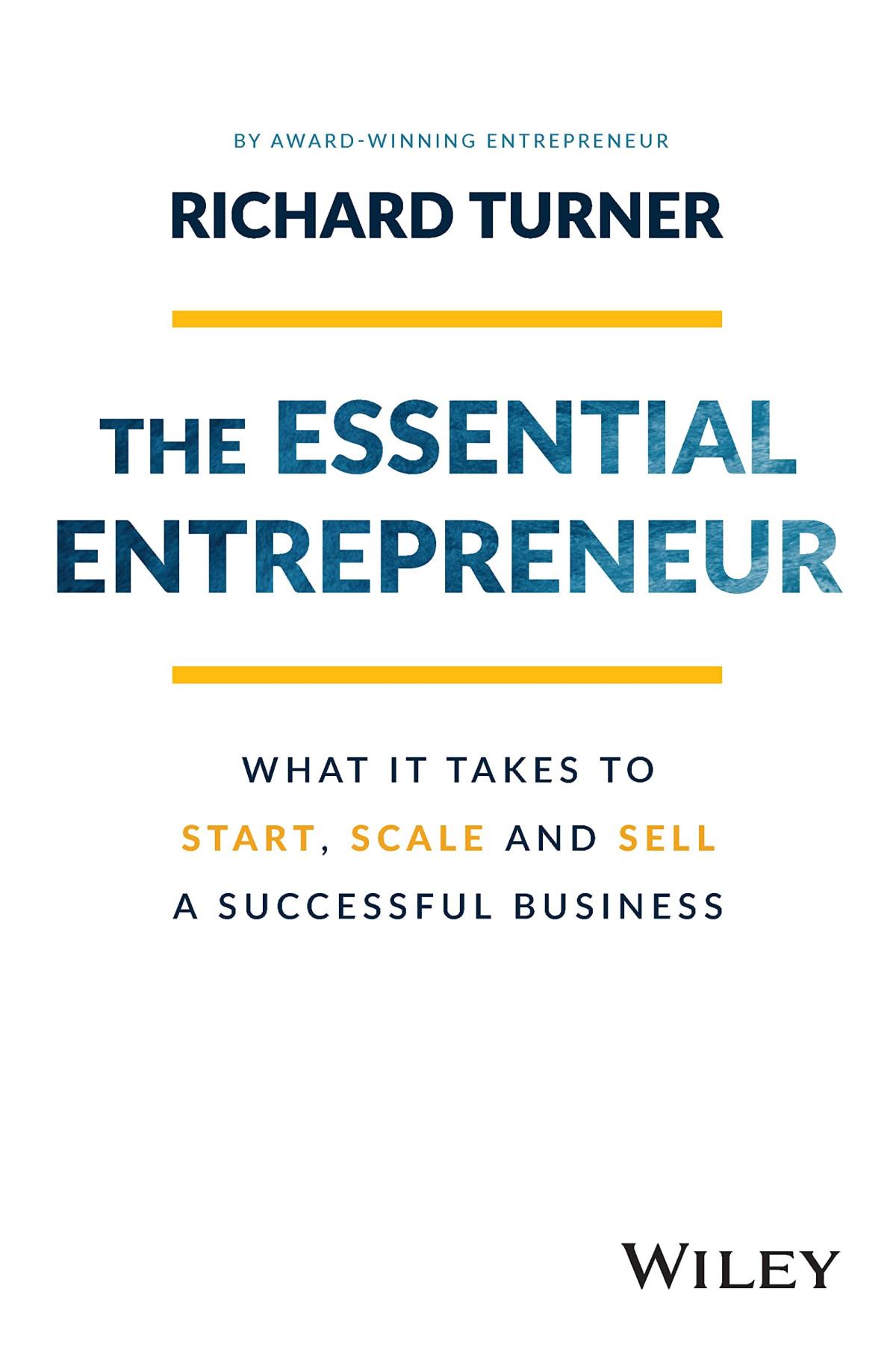
(eBook PDF) The Speaker: The Tradition and Practice of Public Speaking 3rd
https://ebookmass.com/product/ebook-pdf-the-speaker-the-tradition-andpractice-of-public-speaking-3rd/
ebookmass.com
Big Bad Wolf Suleikha Snyder
https://ebookmass.com/product/big-bad-wolf-suleikha-snyder-4/
ebookmass.com
Professional C++ 5th Edition Marc Gregoire
https://ebookmass.com/product/professional-c-5th-edition-marcgregoire-2/
ebookmass.com
Analysis of the Development of Beijing, 2019 1st ed. Edition Zhao Ran
https://ebookmass.com/product/analysis-of-the-development-ofbeijing-2019-1st-ed-edition-zhao-ran/
ebookmass.com
Product Analytics For Dummies®, Amplitude® Special Edition
Brittany Fuller & Anastasia Fullerton
https://ebookmass.com/product/product-analytics-for-dummies-amplitudespecial-edition-brittany-fuller-anastasia-fullerton/
ebookmass.com
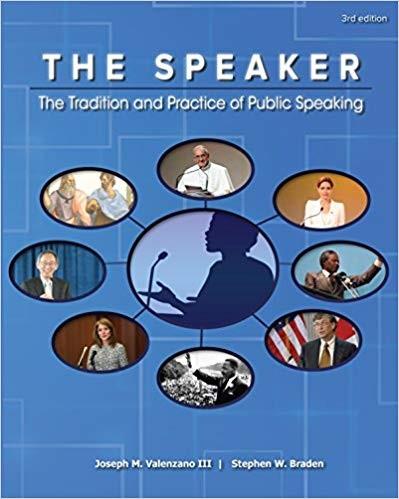
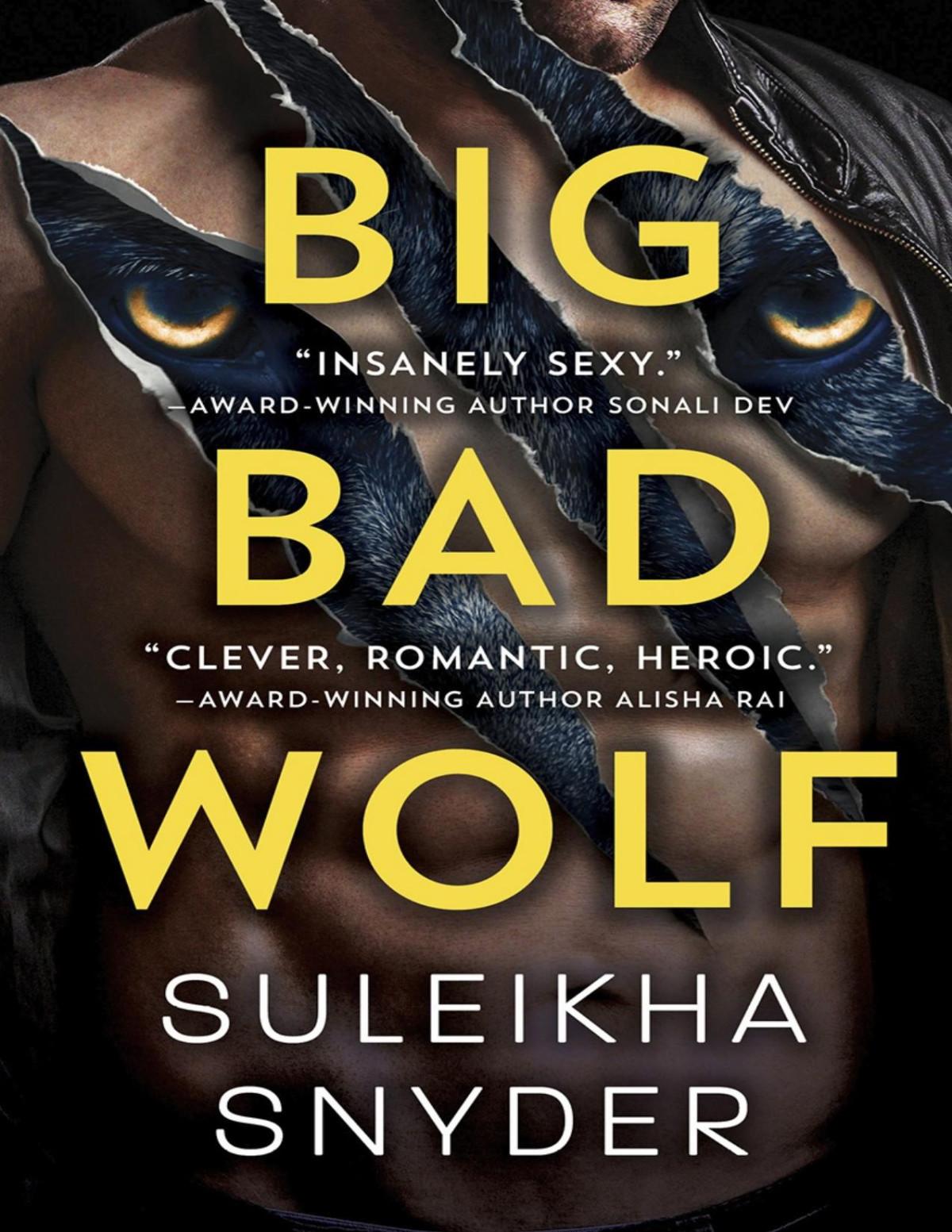
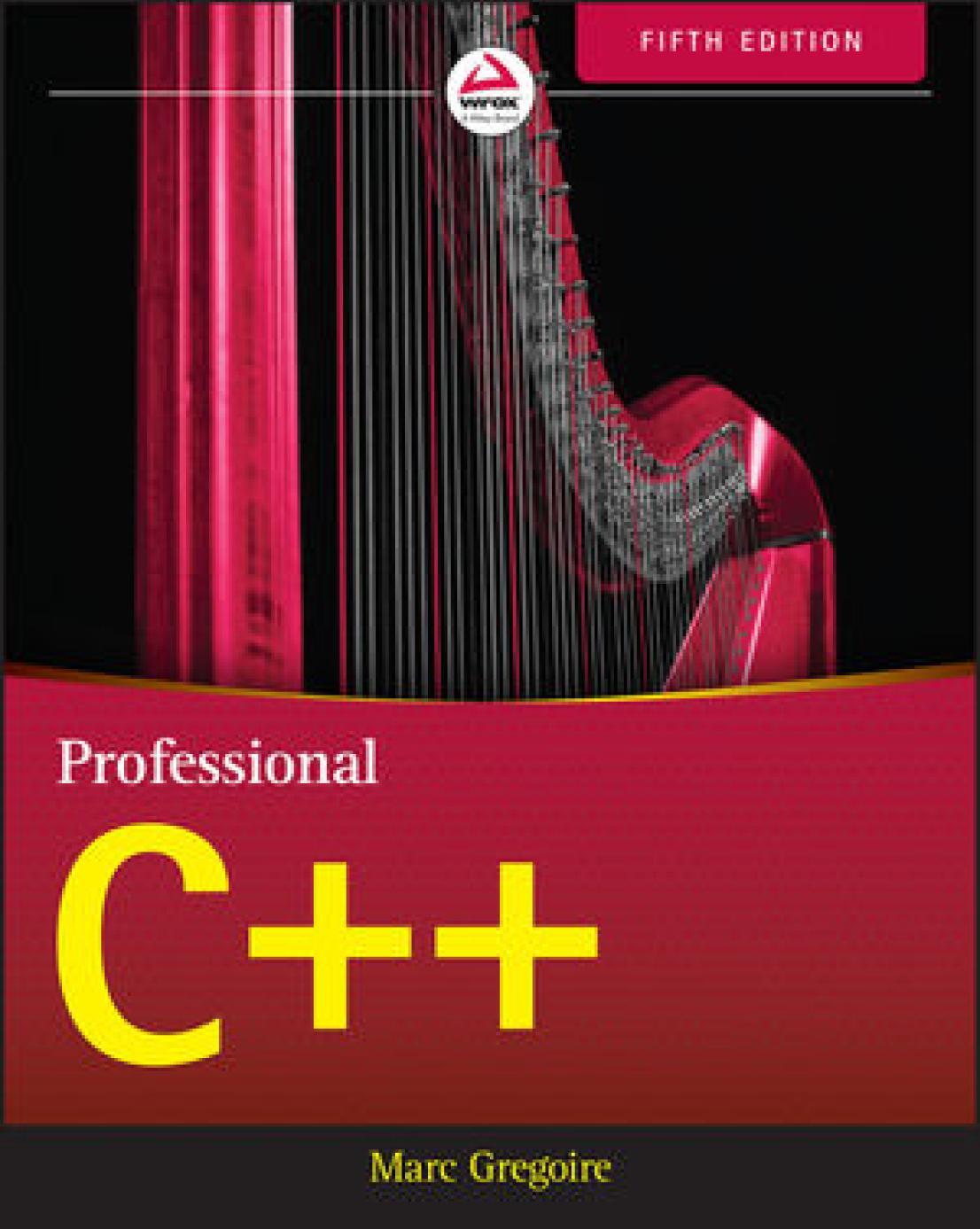
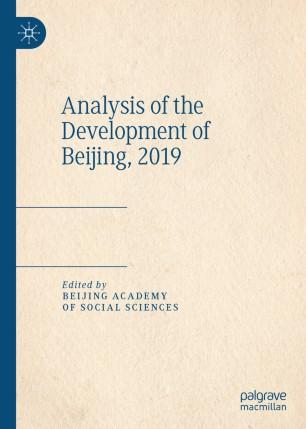
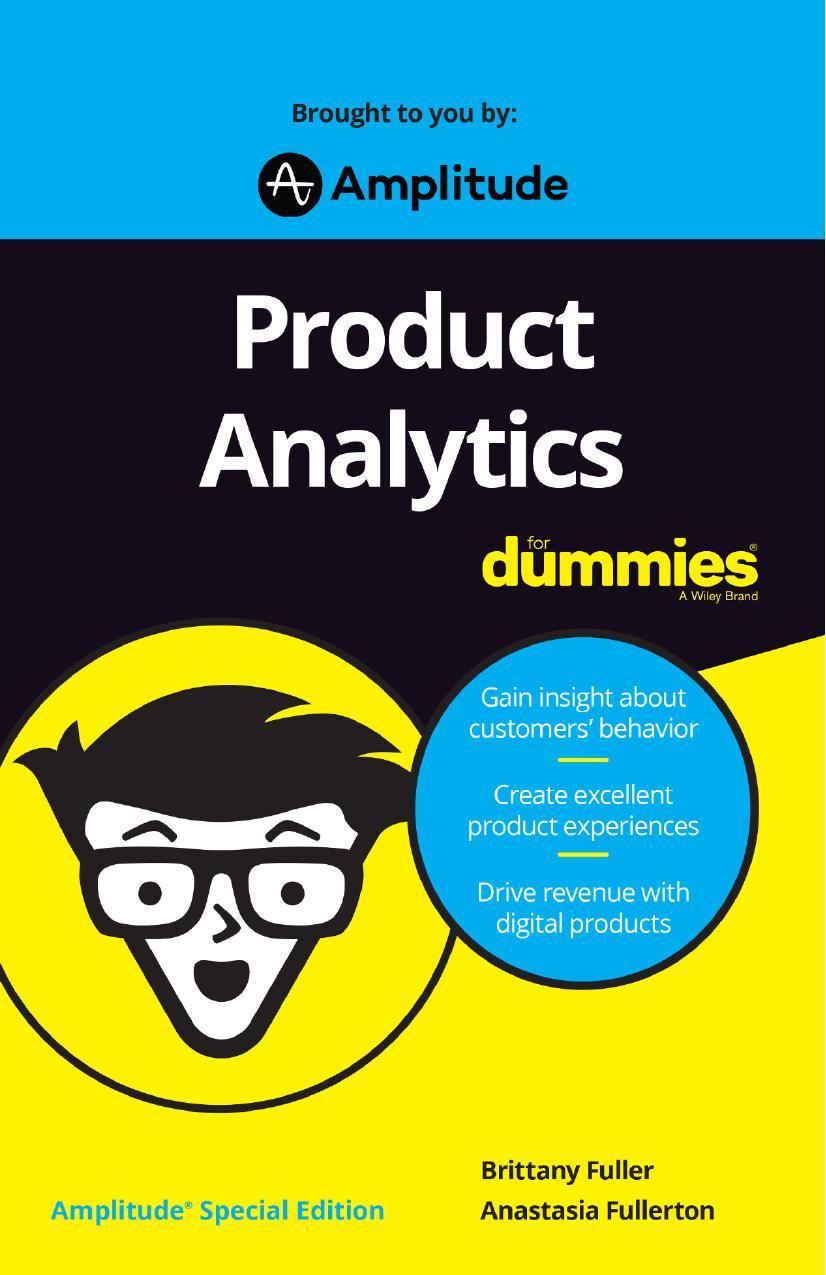
https://ebookmass.com/product/labour-under-corbyn-constraints-onradical-politics-in-the-uk-prapimphan-chiengkul/
ebookmass.com
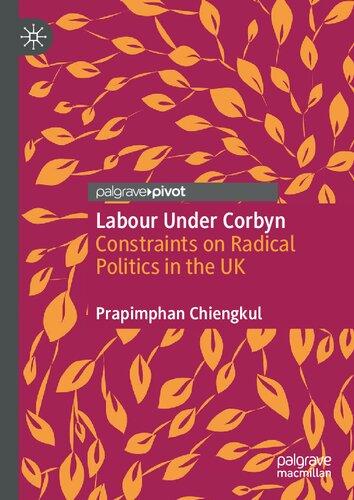
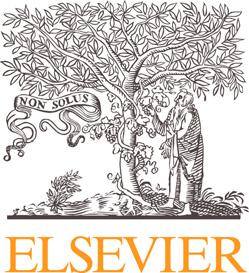
Improved
Contents lists available at ScienceDirect
Electrochimica Acta
journal homepage: www.elsevier.com/locate/electacta
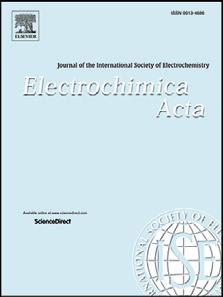
methanol electrooxidation catalyzed by ordered mesoporous Pt-Ru-Ir alloy nanostructures with trace Ir content
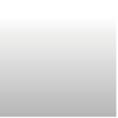
Sabarinathan Ravichandran a,b,1 , Narayanamoorthy Bhuvanendran a,1 , Qian Xu a , Thandavarayan Maiyalagan c,∗ , Huaneng Su a,∗
a Institute for Energy Research, Jiangsu University, 301 Xuefu Road, Zhenjiang 212013, China
b School of Material Science and Engineering, Jiangsu University, 301 Xuefu Road, Zhenjiang 212013, China
c Department of Chemistry, SRM Institute of Science and Technology, Kattankulathur, 603203 Tamil Nadu, India
a r t i c l e i n f o
Article history:
Received 29 June 2021
Revised 12 August 2021
Accepted 22 August 2021
Available online 26 August 2021
Keywords:
Ordered mesoporous catalyst
Oxophilic metals
Trace Iridium
Electronic effect MOR a b s t r a c t
Mesoporous nanostructures of Pt alloy with Ru-Ir as trimetallic electrocatalyst exhibits significant performance for direct methanol fuel cell (DMFC). In this study, the ordered mesoporous nanostructure (OMNs) of Pt-Ru-Ir catalyst was prepared through the KIT-6 mesoporous silica template-assisted chemical reduction method. The OMN of Pt-Ru-Ir possesses high Pt utilization and a low Ir content, resulting in promoted MOR kinetics by the bi-functional mechanism of its oxophilic nature. In addition, the oxides of both Ru and Ir further enhanced the electrocatalytic efficiency of Pt by producing more active sites owing to its ordered mesoporous morphology and bifunctional mechanism. Furthermore, the OMNs of Pt0.7 Ru0.25 Ir0.05 electrocatalyst has a substantial electrochemical surface area (ECSA) of 78.35 m2 g 1 with 1721 mA mg 1 of mass activity, which is comparatively 2-3 times higher than all other catalysts reported here. Notably, the ECSA loss (19.5 %) after 50 0 0 durability cycles was found much lesser than other compositions, Pt/C and Pt0.7 Ru0.3 catalyst owing to the synergistic effect between Pt and Ru with a trace amount of Ir, smaller particle size, and unique ordered mesoporous morphology. Another significant factor is the rate of CO poisoning during MOR, which was found to be much lesser than others for the Pt0.7 Ru0.25 Ir0.05 catalyst (∼0.0047 %) studied by chronoamperometry test. The results indicate that the unique OMNs of Pt-Ru-Ir is an excellent MOR catalyst for DMFC applications.
©2021 Elsevier Ltd. All rights reserved.
1. Introduction
The newly emerged methanol economy is playing an important role as an alternative to conventional energy sources, particularly internal combustion engines, by entering the direct methanol fuel cell, a promising renewable energy system with clear features of eco-friendly operation at low temperature and high energy conversion efficiency [1-3]. The methanol oxidation reaction (MOR) is an important electrochemical reaction that requires an active catalyst material to improve the slow kinetics and multi-step reaction mechanism [4,5]. Pt-based materials are still considered as the most promising MOR catalysts for increasing electrocatalytic performance [5,6]. The key drawback is the high cost of the Pt catalyst, as well as the fact that it rapidly adheres to the poisonous CO intermediate on active sites during the reaction [3,7]. Bimetallic
∗ Corresponding authors.
E-mail addresses: maiyalagan.t@ktr.srmuniv.ac.in (T. Maiyalagan), suhuaneng@ujs.edu.cn (H. Su).
1 Equal contribution.
https://doi.org/10.1016/j.electacta.2021.139148 0013-4686/©2021ElsevierLtd.Allrightsreserved.
(Pt-M) and trimetallic (likely Pt-Ru-M) based alloy catalysts (M= Ir, Pd, Cu, Mn, Fe, and Rh) have been extensively studied for MOR behavior in acidic medium [6,8,9]. A Pt-Ru based catalyst was established to be the most suitable and active catalyst for MOR through a bifunctional mechanism.
Furthermore, the catalyst’s structural morphology is the most influential factor, determining the substantial electrocatalytic efficiency through various aspects of its size, shape, crystalline facets, surface properties, and, most importantly, the synergism between catalyst components [6,10,11]. A wide range of platinum-based multi-metallic alloys with morphology-controlled nanostructures such as core-shell, nanorods, nanowires, nanodendrites, nanocubes, and ordered mesoporous, and others, have been studied extensively for MOR. Depending on the requirements, various types of templates such as PVP, F123, F127, CTAB, P123, F108, SBA-15, and KIT-6 [11-15] are being used to produce these well-designed electrocatalysts. PVP-assisted concave octahedron nanocrystals of PtFeCu were developed by reducing PtCl6 2 , Fe3+ , and Cu2+ ions with ascorbic acid, forming tiny spherical nanoparticles of PtFeCu catalyst for MOR [16]. Pt-M (M= Co, Ni) mesoporous nan-
S. Ravichandran, N. Bhuvanendran, Q. Xu et al.
otubes were prepared using a dual-template approach, i.e., using Te nanowires and F127 micelles as self-sacrificial nanotubes and poreinducing templates to facilitate the construction of mesoporous nanostructure for ORR and MOR [17].
Pt modified by Ru contributes lattice concentration, which increases the activity of multimetallic PtFe@PtRuFe core-shell nanostructures. These nanostructures have excellent MOR efficiency and are resilient to CO poisoning [18] Similarly, the morphologycontrolled Pt-Ru nanodendrites was synthesized by one-pot reduction and exhibits an improved electrocatalytic performance due to the well-spreading atoms of Pt and Ru with an average particle size of 21 nm, reported by Xue et al [5] Tunable hollow Pt@Ru dodecahedra and deformed Pt@Ru dodecahedra catalysts have superior specific activity (SA, 1.61 mA cmPt 2 ) and mass activity (MA, 800 mA mgPt 1 ) compared to Pt/C catalysts due to the bifunctional mechanism [19]. Trimetallic alloy of Pt-Ru-Cu hexapod catalyst was successfully developed by a galvanic replacement through StranskiKrastanov growth mechanism and possesses good activity and stability for MOR with a synergetic effect and retains nearly 27 % of its initial MA after 800 cycles compared to Pt/C (60 %) [11] Surface alloyed Ru with Pt could activate more Pt atoms in the LangmuirHinshelwood (L-H) pathway than core-shell (Ru@PtNWs) and significantly increase the anti-poisoning effect. Li et al. determined that using a Pt-Ru alloy will improve MOR electron transfer kinetics [20] The Pt-Ru-NiTiO3 /C electrocatalyst reported by Thiyagarajan et al. showed high catalytic activity, which was attributable to the synergistic effects of NiTiO3 and the bifunctional mechanism of Ru metal [7]. Esteban et al. reported that the mesoporous Pt/Ru catalysts were synthesized using the F127 copolymer template, and found higher accessibility of active areas for MOR due to the large pore diameter boost the catalyst performance [21]
In order to evaluate the influence of electrode catalytic activity towards MOR, Bhuvanendran et al. reported the effect of Ir material on Ptx Ir/MWCNT catalysts and described the impact of lattice strain [22] The inclusion of Ir in Pt-based bimetal alloy catalysts can be used in terms of bifunctional mechanism due to secondary metals’ oxophilic nature, which makes them affinitive to adsorption of a large number of hydroxyl groups [23,24]. Furthermore, the presence of Ir with Pt catalyst can boost oxidation activity by providing dual-enhanced electronic and CO tolerance compared to PtRu, resulting in improved methanol dehydrogenation. This may be due to the weakening binding energy of Ir metal, which is caused by the large electronic band distance [24] On the other hand, the coexistence of oxophilic metal oxides like RuO2 and IrO2 can increase the utilization of Pt active sites, ultimately increasing catalytic efficiency [22,25]. RuO2 particles are in very close contact with the Pt-Ru catalyst due to the isolation and high use of Ru metal, which could lead to the excellent CO-tolerance, higher activity, and greater stability [26]
Furthermore, RuO2 content has a great influence on Pt/RuO2 for the electrocatalytic methanol oxidation activity due to electron conductivity and electron transfer between Pt and Ru [27] Similarly, the mesoporous shell and hollow structure promote the transport of the mass/charge to the internal surface of the catalysts, allowing for good metal use [28] Hence, the newly developed 3D porous Pt/RuO2 /G demonstrated enhanced catalytic performance in terms of anti-poisoning rate and extended stability against MOR [25] Although Pt, Ru, and Ir are costly precious metals, Pt group metals (PGM) remain widely-used materials and essential catalysts for fuel cell applications. As a result, there is a growing trend toward creating expense new electrocatalysts with less PGM or indeed PGM-free catalysts that may be used without loss of performance by enhancing the utilization rate of noble metals catalysts [22,29].
In this work, trimetallic ordered mesoporous nanostructures of Pt-Ru-Ir as a supportless catalyst were synthesized by chemical re-
duction utilizing KIT-6 as a surfactant and sodium borohydride as a reducing agent. The Pt0.7 Ru0.25 Ir0.05 nanoparticles were applied as an electrocatalyst with an average diameter of 3.59 nm and shown electrooxidation of methanol activity and long-term durability. Moreover, the electrocatalytic activity of the Pt0.7 Ru0.25 Ir0.05 was higher than that of state-of-the-art Pt/C and Pt0.7 Ru0.3 catalysts due to its rich mesoporosity and synergistic impact between Pt, Ru, and trace amounts of Ir.
2. Experimental methods
2.1. Chemicals and materials
Potassium hexachloroplatinate (K2 PtCl6 , 99%), Ruthenium (III) chloride hydrate (RuCl3 •xH2 O, 99.9%), and Hydrogen hexachloroiridate (IV) hydrate (H2 IrCl6 •xH2 O, 99.9%) were purchased from Strem Chemicals. Pluronic P123 (PEG-PPG-PEG), n-Butyl alcohol, Tetraethyl orthosilicate (TEOS), Ethanol (99.9%), Sodium borohydride (NaBH4 ), and 5 wt. % of Nafion solution was acquired from Sigma- Aldrich. Pt/C catalyst (20 % Pt loading) was obtained from Sainergy Fuel Cell India Private Limited and Methanol, Sulfuric acid (H2 SO4, 37 wt. %), hydrochloric acid (HCl, 38 wt. %), and hydrofluoric acid (HF, 10 wt. %) solution were obtained from Rankem, India.
2.2. Synthesis of mesoporous silica KIT-6
A typical synthesis of ordered mesoporous silica template (KIT6) was followed using previously published literatures [30] 6 g of Pluronic P123 was mixed into 217 g of double-distilled (DD) water and then mixed well by magnetic stirring for 30 min at room temperature. After that, 6 g butanol and 1.628 g HCl were added to the Pluronic P123 aqueous mixture with vigorous stirring. Once the above mixture was fully dissolved, 12.9 g TEOS was added and stirred for 24 hours at 35 °C. Then, the reaction mixture was transferred to a 250 ml Teflon stainless-steel autoclave and heated to 100 °C for 24 h. The final product was obtained by centrifugation at 40 0 0 rpm and washed with water and ethanol several times and dried overnight at 60 °C. The collected white colour powder was distributed in a mixture of 300 ml ethanol and 6.2 ml HCl under stirring for 15 min at room temperature. Finally, the yield was washed numerous times with water and ethanol before being dried at 60°C and calcined for 5 h at 550°C.
2.3. Synthesis of ordered mesoporous nanostructures (OMNs) of Pt-Ru-Ir catalysts
Pt0.7 Ru0.25 Ir0.05 mesoporous were synthesized as follows: An aqueous solution of K2 PtCl6 (25 mg, 0.0208 M), RuCl3 •xH2 O (32 mg, 0.046 M), and H2 IrCl6 •xH2 O (8 mg, 0.0527 M) were mixed in a 50 ml beaker under continuous stirring for 1 h. In another 50 ml beaker, 360 mg of KIT-6 dissolved in 12.41 ml DD water. Then, the Pt-Ru-Ir homogenous water mixture was gradually applied to the KIT-6 dispersion and stirred for 12 h. After that, 10 ml of freshly prepared 0.1 M NaBH4 aqueous solution added dropwise under vigorous stirring at room temperature for 1 h. Finally, Pt-Ru-Ir/KIT-6 mixture was dried in the oven for 6 h at 100 °C, and the dried sample was dissolved in 10 wt. % HF solution to remove KIT-6 template by acid etching process overnight with continuous stirring. Later, it was washed several times with water and ethanol and before being dried for 6h at 80 °C. Similarly, other compositions of Pt0.7 Ru0.2 Ir0.1 and Pt0.7 Ru0.15 Ir0.15 catalyst were prepared by following the above synthesis procedure with an appropriate percentage of elemental compositions. All the catalysts were prepared based on the atomic weight of each element. For Pt-Ru catalyst, the same procedure was adopted without Ir content and the composition was fixed as Pt0.7 Ru0.3.
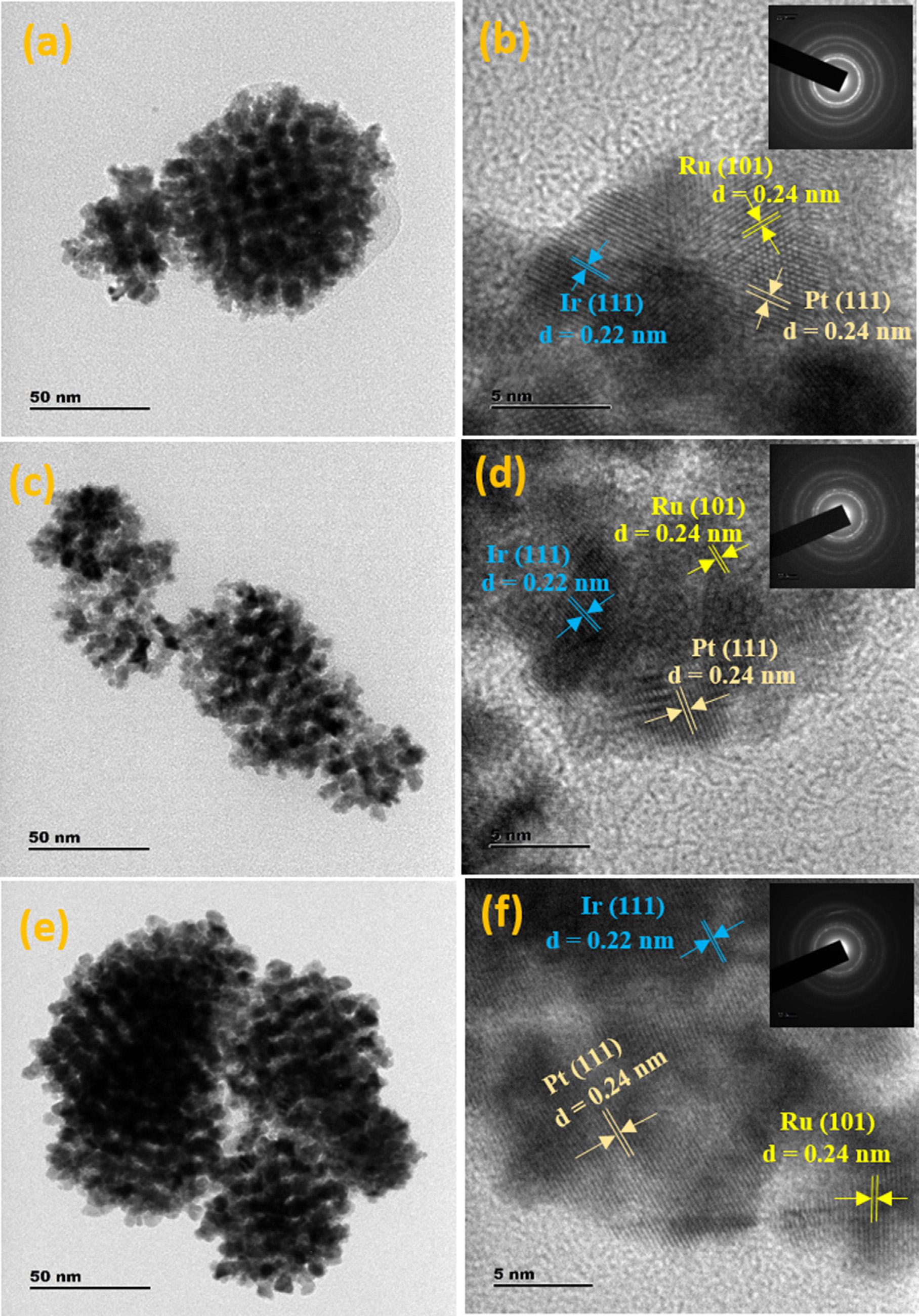
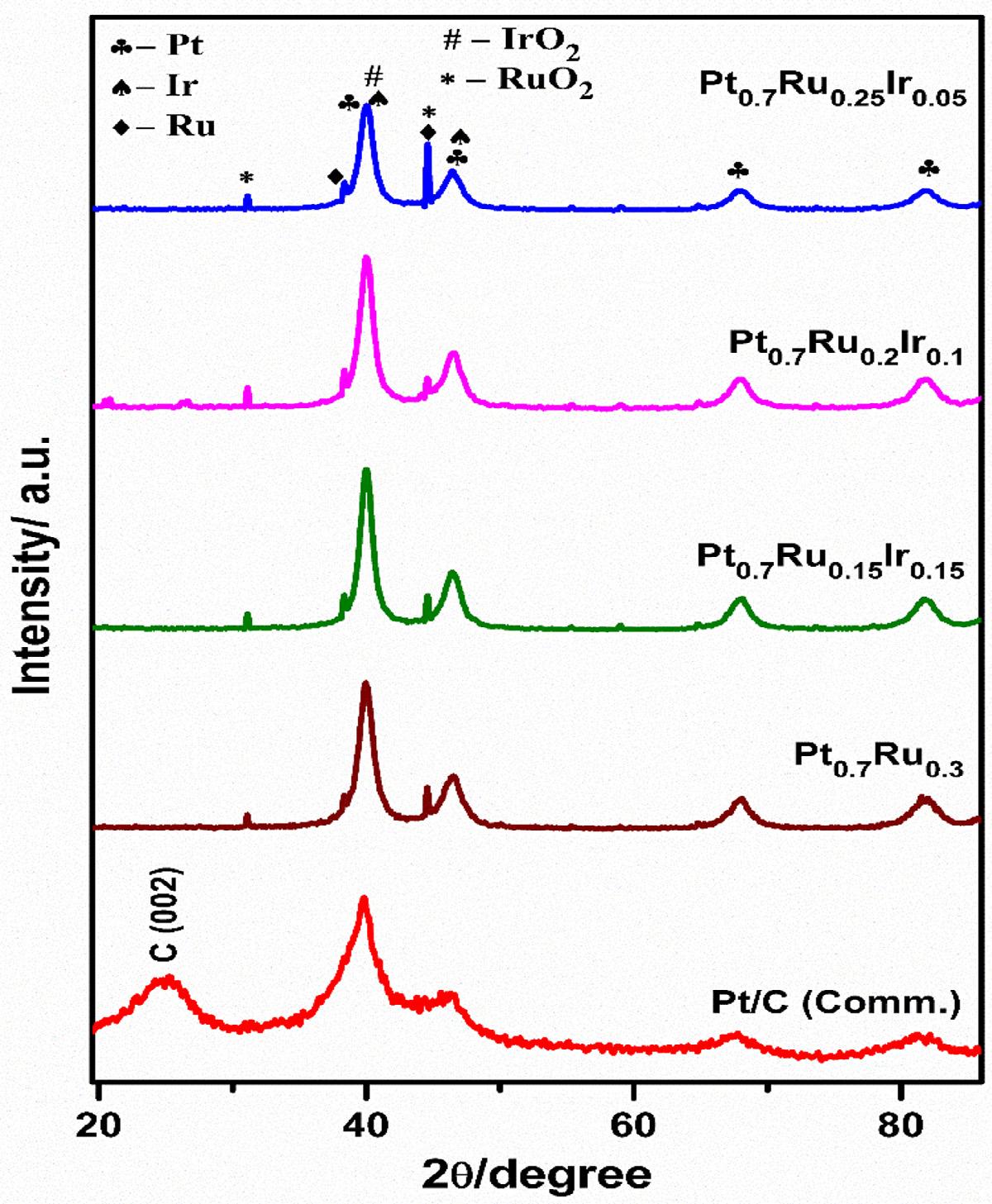
2.4. Materials characterization
The morphology of Pt-Ru-Ir catalysts was analyzed using a scanning electron microscope (SEM- FEI Quanta, 200) and) and high-resolution transmission electron microscope (HRTEM, JEOL, Japan). X-ray diffraction (XRD) examination was performed using BRUKER instrument with Cu Kα radiation from 10 - 90° , X-ray photoelectron spectroscopy (XPS) was carried out using physical electronics (PHI 50 0 0 VersaPobe III) to estimate the elemental composition. Inductively coupled plasma atomic emission spectroscopy (ICP-AES) was used to estimate the elemental composition. Surface area, size, and volume of pores were measured from Brunauer–Emmett–Teller ((BET) method using by N- adsorption and desorption isotherm (Quantachrome Autosorb AS-1Version-1.55).
2.5. Electrochemical measurements
The electrochemical tests were conducted on a three-electrode electrochemical cell coupled with an electrochemical workstation (AUTOLAB, The Netherlands). Rotating disk electrode (GC-RDE) was used as a working electrode, platinum wire and Ag/AgCl (3 M KCl) were used as a counter and reference electrode respectively. All the potential was converted against reversible hydrogen electrode (RHE) for universality convenience. For each electrocatalyst, a homogeneous catalyst ink solution was prepared in the following manner. 2 mg catalyst was dispersed in 4220 μl of solvent mixture (4.2 ml C2 H5 OH + 20 μl 5 wt. % Nafion) using ultrasonication until homogeneity was achieved. Then, 10 μl of catalyst ink
(16. 92 μg cm 2 of Pt) was coated on the GC-working electrode and dried at room temperature. Initially, the cyclic voltammetry (CV) was measured in N2 saturated 0.5 M H2 SO4 for basic studies and then in 0.5 M H2 SO4 +0.5 M CH3 OH for MOR at a sweep rate of 0.1 V s 1 The durability test was carried out between 0.4 V and 1.2 V vs. RHE at a scan rate of 0.1 V s 1 in 0.5 M H2 SO4 +0.5 M CH3 OH solution up to 50 0 0 potential cycles. The Chronoamperometry test was recorded at 0.94 V vs RHE under similar conditions.
From the basic CV studies, electrochemical active surface area (ECSA) was calculated using the charge associated with hydrogen adsorption/desorption peak observed between 0.03-0.2 V vs. RHE by applying the following equation [31].
ECSA = QHdes 210 × m (1)
where, QHdes is the charge of H-desorption from CV (μC cm 2 ), m is the mass of Pt-Ru-Ir metal loading (16.92 μg cm 2 ) over the working electrode, 210 μC cm 2 is the monolayer of hydrogen desorption on a polycrystalline Pt surface [17]
3. Results and discussion
Figure 1 shows the TEM and HRTEM images of Pt-Ru-Ir catalysts, which revealed a unique morphology of ordered mesoporous nanostructures. The TEM images (Figure 1a, 1c, and 1e) of all three different compositions of mesoporous Pt-Ru-Ir catalysts and the average particle size was estimated using ImageJ software and
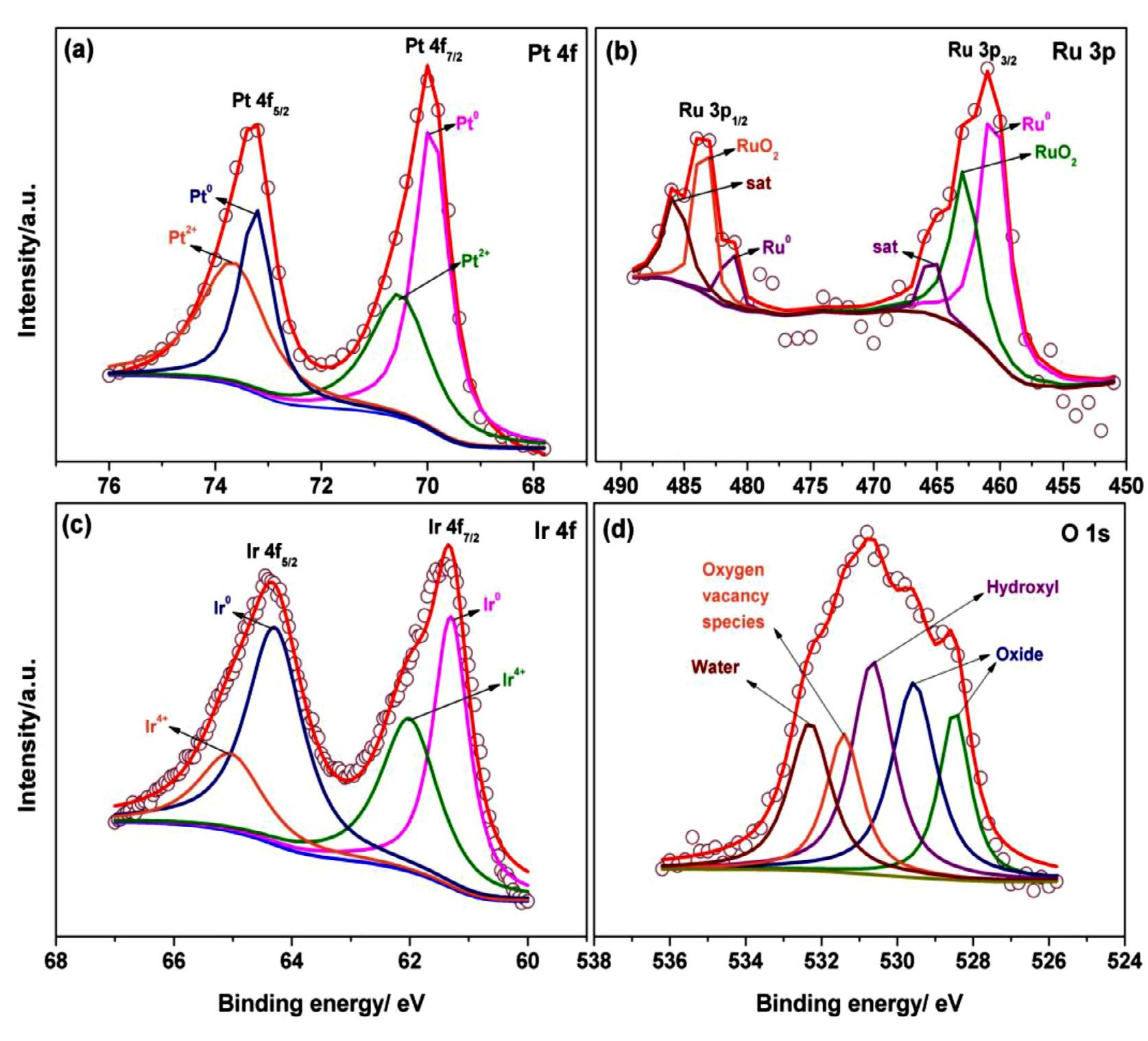
Fig. 3. XPS patterns of Pt0.7 Ru0.25 Ir0.05 catalyst (a) Pt 4f region, (b) Ru 3p region, (c) Ir 4f region, and (d) O1s region.
found to be ∼4.5 nm. The lattice fringes with d-spacing values of 0.24 nm for Pt (111), 0.24 nm for Ru (101), and 0.22 nm for Ir (111) crystalline facets are well recognized from the respective HRTEM images (Figure 1b, 1d, and 1f), which are in good accordance with the reported literature [22,32-34]. Similarly, the low magnification TEM images were provided in supporting information Figure S1, which reveals that the majority of nanoparticles were formed in ordered mesoporous nanostructure, despite the fact that the staking of mesoporous metal nanostructures and their density causes the materials to appear agglomerated. Whereas, the FESEM images shown in Figure S2a, S2c, S2e, and S2g display the clustered morphology of all the three Pt-Ru-Ir and Pt-Ru catalysts, and the elemental composition was tabulated as an insert of Figure S2b, S2d, S2f and S2h for each of catalysts, obtained from the SEM-EDX analysis [25,35]. These values are found to be quite comparable to the catalyst elemental composition as established during the synthesis. Furthermore, the Pt, Ir, Ru, and O elements have been distributed uniformly in Pt0.7 Ru0.25 Ir0.05 catalyst, and the alloy formation of Pt, Ir, and Ru trimetallic nanoparticles was confirmed using FESEMEDX mapping (Figure S3a-S3e). The selected area electron diffraction (SAED) patterns were obtained to the corresponding catalysts and showed as an insert of Figure 1b, 1d, and 1f. The lattice fringes were observed with diffused and intense points located in SAED rings for all three Pt-Ru-Ir catalysts, revealing the crystalline nature and being consistent with XRD results [36]. Additionally, ICP-AES was used to estimate the composition of Pt0.7 Ru0.25 Ir0.05 , and the percentage of Pt, Ru, and Ir were determined to be 68.4 %, 25.5%,
and 6.1 %, respectively. These values are well associated with the SEM-EDX results as shown in Figure S2.
The crystal structure and alloy formation of Pt-Ru-Ir catalysts for various elemental compositions were investigated using powder X-ray diffraction (XRD) analysis [37] Figure 2 compares the diffraction peaks of the respective Pt-Ru-Ir catalysts to Pt-Ru and Pt/C (Comm.), showing that the obtained XRD patterns have predominant peaks attributing to the Pt crystalline planes with some additional peaks at lower diffraction angles [38] The characteristic peaks can be assigned to the (111), (200), (220), and (311) diffraction planes corresponding to the face-centered cubic (fcc) structure of the Pt metal for all the three compositions of PtRu-Ir and Pt-Ru catalysts, including Pt/C (Comm.) [7,20]. When compared to standard Pt/C (Comm.), all the three Pt-Ru-Ir display a positive shift towards the higher diffraction angle, in which the Pt0.7 Ru0.25 Ir0.05 shows a slightly higher degree of peak shifting than other two compositions. Similarly, as referred to the Pt/C (Comm.), the Pt0.7 Ru0.3 catalyst exhibits a positive shift. The peak shifting towards higher diffraction angles is a well-identified phenomenon that endorses the formation of Pt alloys with Ru and Ir in both Pt-Ru-Ir and Pt-Ru catalysts [36]
In addition, the oxides of Ir and Ru diffraction peaks can be observed at 34.54° , 40.26˚ and 44.67˚ assigned to (101), (200), and (120) diffraction planes respectively, with some of them appearing as shoulder peaks due to overlap with the high intensity of Pt (fcc) crystalline planes. Hence, the presence of IrO2 and RuO2 has been substantiated by the corresponding peaks as stated in the lit-
Table 1
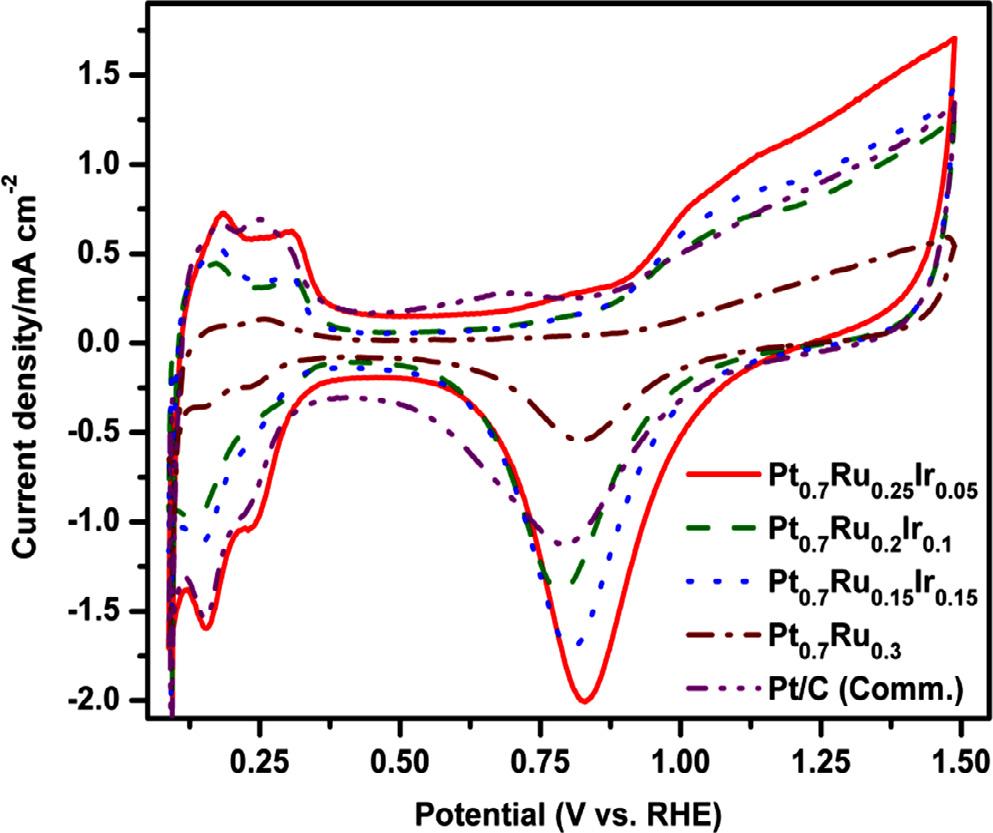
Fig. 4. Cyclic voltammograms recorded in N2 - saturated 0.1 M H2 SO4 electrolyte at 0.1 V s 1 scan rate for
and Pt/C (Comm.) catalysts.
erature [26,39], neither any specific peaks for metallic Ru and Ir have been observed. Furthermore, the diffraction peak positions for RuO2 and IrO2 tend to be very close and cannot be distinguished, thus could be due to the similar structure and lattice parameters [40] The intensity of shoulder peaks observed at 40.26˚ , 44.67˚ with (200) and (120) planes was found to be significant and steep for Pt0.7 Ru0.25 Ir0.05 catalyst as compared to the other compositions of Pt-Ru-Ir and Pt0.7 Ru0.3 catalysts, implying strong lattice contraction by intra-metallic interaction between Pt, Ru, and Ir [22]. According to the XRD results, the significance of RuO2 and IrO2 along with active Pt could be attributed as an excellent booster for oxygen evolution (producing OH by dissociation of water), depicting as more beneficial to accelerate MOR activity [22,27].
The crystallite size of the OMNs Pt-Ru-Ir, Pt-Ru, and Pt/C (Comm.) catalysts was calculated using the Scherrer equation for the predominant (111) crystalline plane. The crystallite size was found to be 3.59, 3.75, 3.67, and 4.38 nm for Pt0.7 Ru0.25 Ir0.05 , Pt0.7 Ru0.2 Ir0.1 , Pt0.7 Ru0.15 Ir0.15 , and Pt0.7 Ru0.3 catalysts, respectively. Whereas, the crystallite size of Pt/C (Comm.) was about 2.92 nm. Similarly, the d-spacing and lattice constant (a) values for all the catalysts were determined by applying the following equations, (1) & (2):[41]
d = nλ/sinθ (2)
a = d × h2 + k2 + l 2 (3)
where, λ is the wavelength of incident radiation (λ = 1.5418 ˚ A), d is the spacing of the crystal planes, n is an integer, θ is the X-ray diffraction angle and h, k, l are the miller indices of the particular crystalline plane, (here, h=k=l=1). In Table S1, the structural parameters of all the catalysts from XRD and TEM analysis were
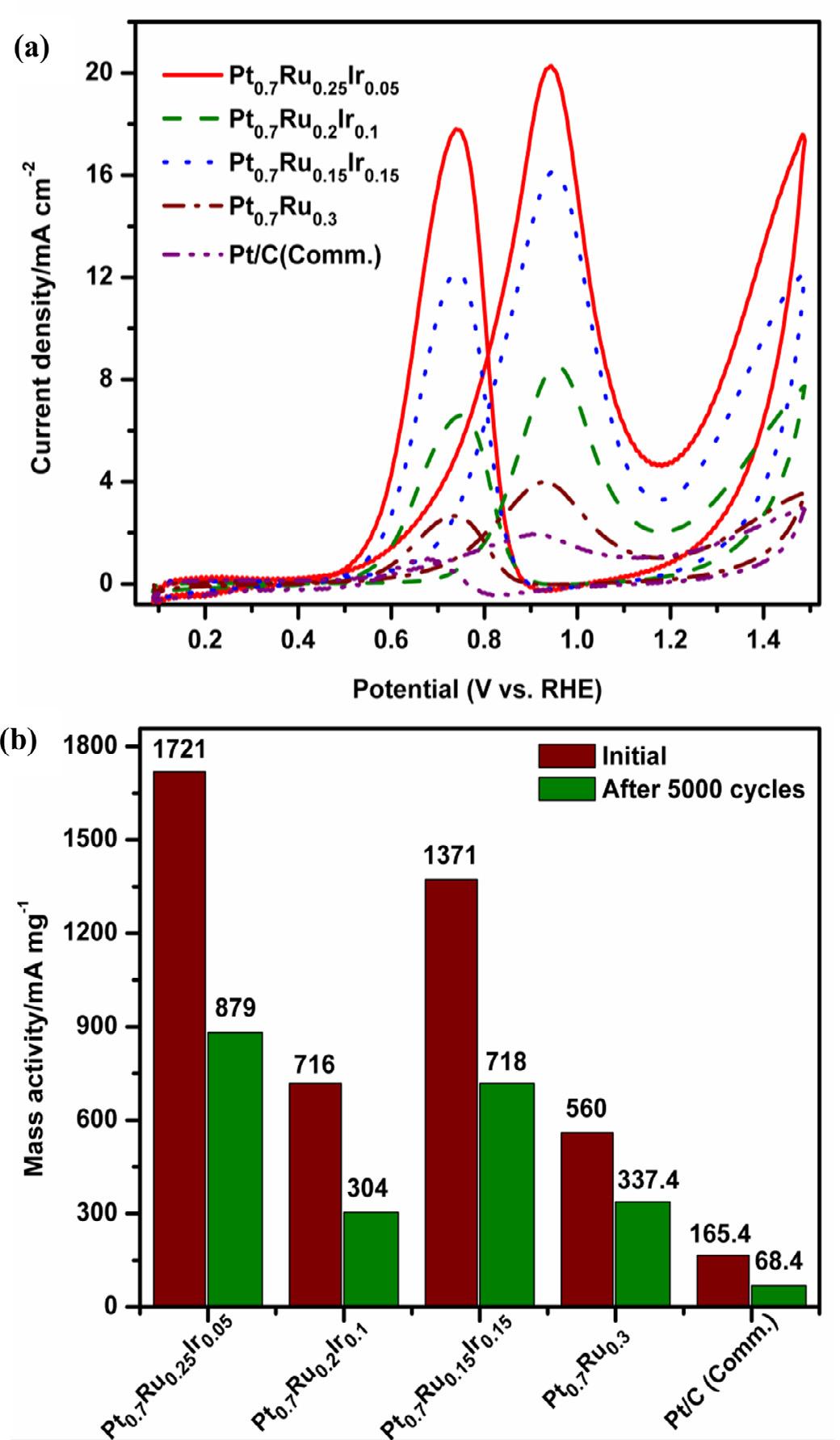
Fig. 5. (a) Cyclic voltammograms of methanol oxidation at 0.1 V s 1 scan rate in N2 saturated 0.5 M methanol + 0.5 M H2 SO4 electrolyte, (b) comparison of Mass activity at peak potentials for Pt0.7 Ru0.25 Ir0.05 , Pt0.7
,
, and Pt0.7 Ru0.3 and Pt/C (Comm.) catalysts.
summarized. The d-spacing value of Pt0.7 Ru0.25 Ir0.05 was found to be 2.2473 A, which is comparatively smaller than Pt0.7 Ru0.2 Ir0.1 , Pt0.7 Ru0.15 Ir0.15 , Pt0.7 Ru0.3 (2.2576 ˚ A), and Pt/C (Comm.) (2.2673 ˚ A) catalysts. Whereas, the similar trend was observed for lattice constant values in this following order as Pt0.7 Ru0.25 Ir0.05 (3.8925 ˚ A) <Pt0.7 Ru0.2 Ir0.1 (3.9103 ˚ A) <Pt0.7 Ru0.15 Ir0.15 (3.9103 ˚ A) <Pt0.7 Ru0.3 (3. 9103 A) <Pt/C (Comm.) (3.9273 A). Based on the lattice constant values, the percentage of compressive strain was calculated and about -0.886 % for Pt0.7 Ru0.25 Ir0.05 catalyst, which is nearly two
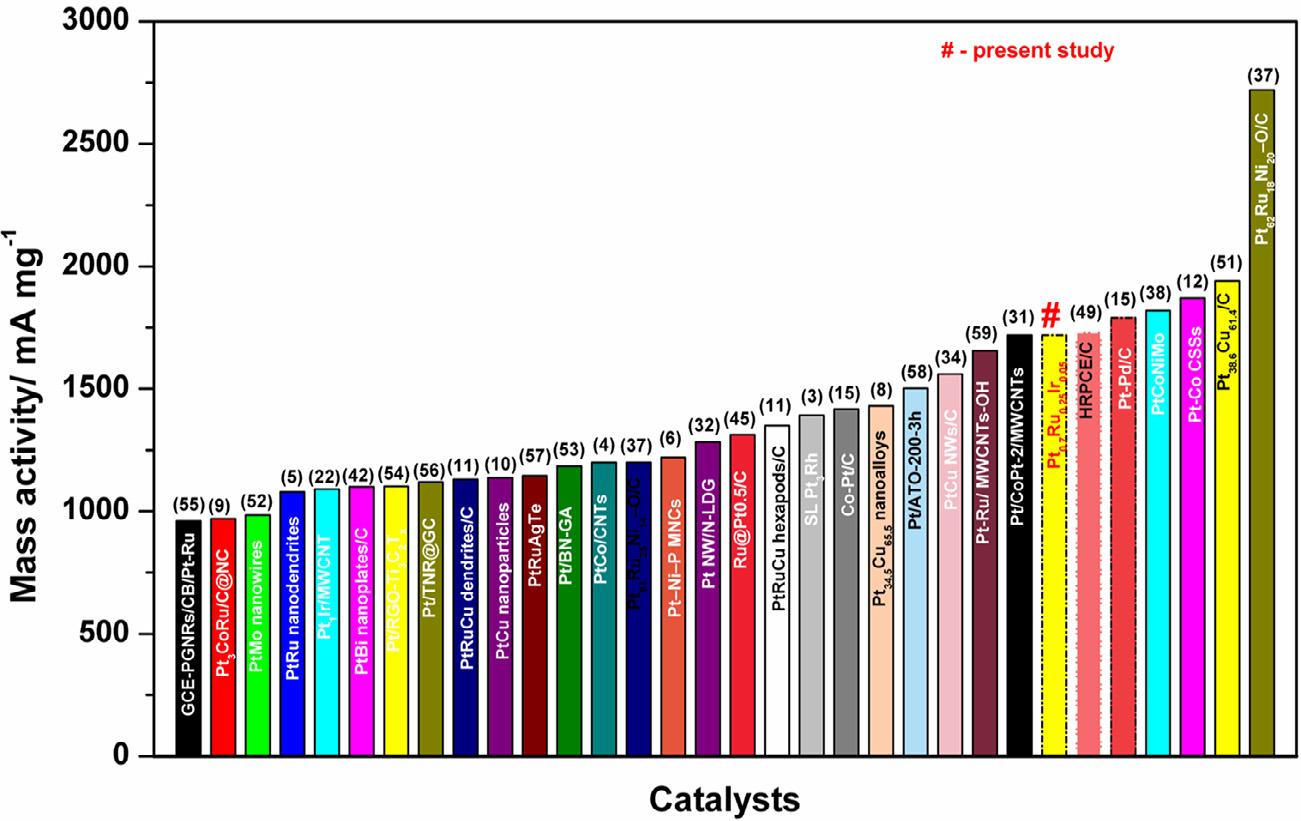
times higher than all other catalysts might be due to the impact of trace Ir content and its synergism with Pt and Ru elements.
The BET-specific surface area (SSA) and mesoporous behavior of Pt0.7 Ru0.25 Ir0.05 catalyst was analyzed using N2 adsorptiondesorption isotherm as shown in Figure S4 (Supporting information). The SSA was found to be 118.3 m² g 1 with an average diameter of the pore size of about 2.65 nm, using the Barrett-JoynerHalenda (BJH) process and the adsorption isotherm (inset of Figure S4) [36]. As a result, the larger SSA might well be attributed to the unique characteristics of the ordered mesoporous morphology of the Pt-Ru-Ir catalyst, and the obtained values are in strong accordance with the template’s wall thickness (KIT-6) and published literature [35]
The electronic state of elements and surface composition of the Pt0.7 Ru0.25 Ir0.05 and Pt0.7 Ru0.3 catalysts were scrutinized using Xray photoelectron spectroscopy (XPS). The deconvoluted XPS peaks of Pt 4f, Ru 3p, Ir 4f, and O 1s elements for both Pt0.7 Ru0.25 Ir0.05 , Pt0.7 Ru0.3 catalysts are shown in Figure 3a-d and Figure S5a-c, respectively. The deconvoluted Pt 4f spectra of the Pt0.7 Ru0.25 Ir0.05 catalyst (Figure 3a) represents a combination of doublets with Pt 4f5/2 and Pt 4f7/2 peaks at 73.20 eV and 70.02 eV corresponding to metallic state (Pt0 ) and another set of doublets of 73.70 eV (4f5/2 ) and 70.57 eV (4f7/2 ) corresponding to divalent state (Pt2+ ), might be of Pt-O or Pt-OH formations [22,25,42]. As like that of the Pt0.7 Ru0.3 catalyst (Figure S5a) exhibit a Pt 4f doublets at 73.20 eV (4f5/2 ) and 69.80 eV (4f7/2 ) corresponding to Pt4+ and Pt2+ respectively, with a negative shift of 0.22 eV for Pt 4f7/2 compared to Pt0.7 Ru0.25 Ir0.05 catalyst [20]
Figure 3b shows that the Ru 3p has asymmetric peaks with broad tails at 483.0 eV and 461.0 eV for 3p1/2 and 3p3/2 , respectively, assigned to the metallic (Ru0 ) and ionic (Ru4+ ) states [4345], with some satellite peaks in the higher binding energy region for the Pt0.7 Ru0.25 Ir0.05 catalyst. Similarly, the Pt0.7 Ru0.3 catalyst (Figure S5b) has the respective peaks of Ru 3p at very close binding energy values of 461.01 eV (3p3/2 ) and 482.7 eV (3p3/2 ) to the Pt0.7 Ru0.25 Ir0.05 catalyst. The XPS spectra for O 1s shows a broad single peak with multiple deconvoluted peaks assigned to a variety of oxygen states such as oxide (528.4 & 529.6 eV), hydroxide (530.6 eV), the water molecule (532.4 eV), and vacant oxygen (531.4 eV) as shown in Figure 3d [44,46]. Relatively similar characteristics of O 1s XPS peaks were observed for the Pt0.7 Ru0.3 catalyst and shown in Figure S5c. Furthermore, the vacant oxygen from O 1s XPS suggests potential electron transfer by the overlapping of Pt
and Ru, as well as a slight negative change of BE (0.22 eV) for Pt 4f compared to pristine Pt [46], revealing that there could be more active sites for MOR.
Furthermore, the deconvoluted Ir 4f spectra (Figure 3c) showed a doublet of Ir 4f7/2 and 4f5/2 at 61.35 eV and 64.35 eV, referring to the metallic and tetra valence states of iridium, respectively [22,39]. The outer catalyst surface is likely to have interconversion of Ir redox states in the form of oxide/hydroxide species, which could enable facile electron transfer at the interface of active sites during methanol oxidation [47]. According to the bifunctional mechanism, the involvement of hydroxide species either from Ru and Ir may actively participate in removing the strongly adsorbed CO species during methanol dehydrogenation. As a consequence, combining two active oxophilic elements (such as Ru and Ir) with Pt, in the form of oxides could enhance methanol oxidation kinetics while also contributing to improved stability under durability test conditions [48] Based on the XPS study, the elemental composition (in atomic %) of Pt0.7 Ru0.25 Ir0.05 catalyst was estimated to be 49.4 % Pt, 18.1 % Ru, 3.1 % Ir, and 29.4 % oxygen, while Pt0.7 Ru0.3 catalyst had 51.4 % Pt, 20.3 % Ru, and 28.3 % oxygen.
Using steady-state voltammetry techniques, the electrocatalytic efficiencies of OMNs of Pt-Ru-Ir, Pt-Ru, and industrial Pt/C catalysts have been extensively investigated for MOR. The electrochemical characterization of the catalysts was examined by recording the CV profiles in N2 saturated 0.5 M H2 SO4 at 0.1 V s 1 [17] In Figure 4, the CV profiles of all three Pt-Ru-Ir with Pt-Ru and Pt/C (Comm.) catalysts, exhibit a typical butterfly pattern with hydrogen adsorption/desorption (Hads /Hdes ) and oxide formation/reduction peaks at low and high potential regions, respectively [3,20]. Both Pt-Ru-Ir compositions and Pt-Ru catalysts have a CV pattern that is close to that of Pt/C (Comm.), which clearly indicates that surface reactions were dominated by Pt active sites. The electrochemically active surface area of Pt0.7 Ru0.25 Ir0.05 was estimated from the Hads/Hdes peak area and found to be around 78.35 m2 g-1 as shown in Table 1, which is significantly higher than other compositions of Pt0.7 Ru0.2 Ir0.1 (43.2 m2 g-1 ) and Pt0.7 Ru0.15 Ir0.15 (43.03 m2 g-1 ) catalysts [22]. The ECSA of the Pt0.7 Ru0.3 catalyst is nearly three times lower (25.8 m2 g-1 ), and the Pt/C (Comm.) (77.01 m2 g-1 ) is nearly equal to that of the Pt0.7 Ru0.25 Ir0.05 catalyst. The particle size distribution and elemental composition of the Pt0.7 Ru0.25 Ir0.05 catalyst contribute to a higher ECSA.
The MOR activity of OMNs of Pt-Ru-Ir, Pt-Ru, and Pt/C (Comm.) catalysts were determined by CV in N2 saturated 0.5 M
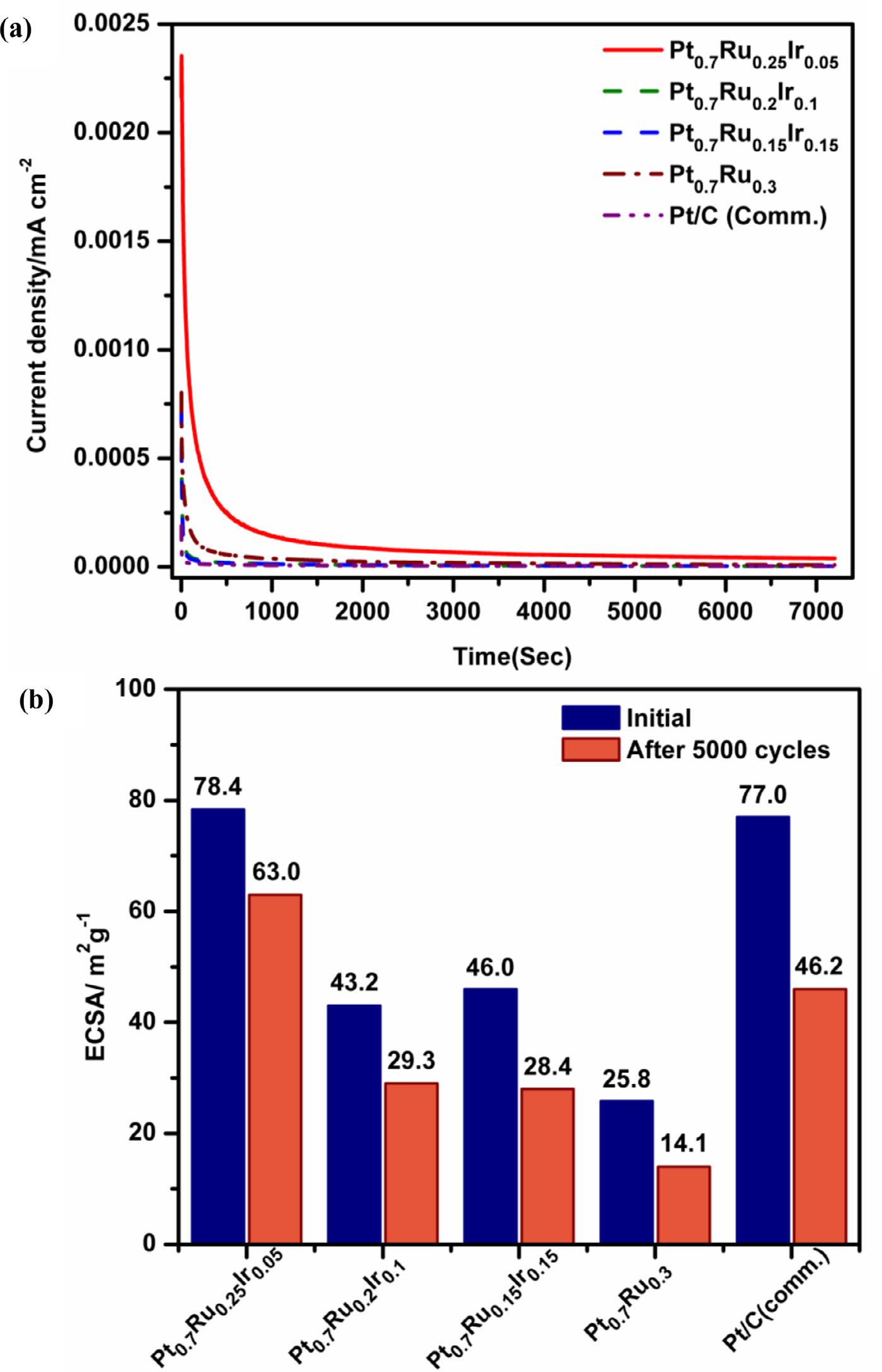
Fig. 7. (a) Chronoamperometry curves of Pt-Ru-Ir, Pt-Ru and Pt/C (Comm.) catalysts recorded at 0.65 V vs. RHE, and (b) bar chart of ECSA before and after the durability test.
methanol + 0.5 M H2 SO4 electrolyte at a scan rate of 0.1 V s 1 (Figure 5a) [5,49]. As can be observed from the MOR-CV profiles, the Pt0.7 Ru0.25 Ir0.05 catalyst has superior methanol oxidation performance, i.e., the onset potential was around 0.45 V and the forward peak current density was about 20.38 mA cm 2 at 0.94 V, which can be ascribed to the dehydrogenation of methanol. During the reverse potential scan, a second oxidation peak was observed with a current density of 17.82 mA cm 2 at 0.74 V, implying that the surface adsorbed intermediate fragmentations were further oxidized. Notably, all three Pt-Ru-Ir catalyst compositions have relatively high MOR activity than Pt0.7 Ru0.3 and Pt/C (Comm.) catalysts, which could be associated with the synergistic impact of Pt with
Ru and Ir elements. Furthermore, as described in many works of literature, the presence of Ru-oxides and Ir-oxides plays a very important role in the oxidation reaction of methanol by restoring the active Pt sites through a bifunctional mechanism [20,50]. As a result, it was clearly demonstrated and addressed using XRD and XPS results for the respective Pt-Ru-Ir catalysts.
In terms of mass activity (Figure 5b), the Pt0.7 Ru0.25 Ir0.05 has a superior magnitude of 1721 mA mg 1 compared to Pt0.7 Ru0.2 Ir0.1 (716 mA mg 1 ), Pt0.7 Ru0.15 Ir0.15 (1371 mA mg 1 ), and Pt0 7 Ru0.3 (560 mA mg 1 ) catalysts, which is potentially competing with other reported benchmark MOR catalysts from reported literatures [3-6,8-12,15,22,31,32,34,37,38,42,45,49,51-59] as presented in
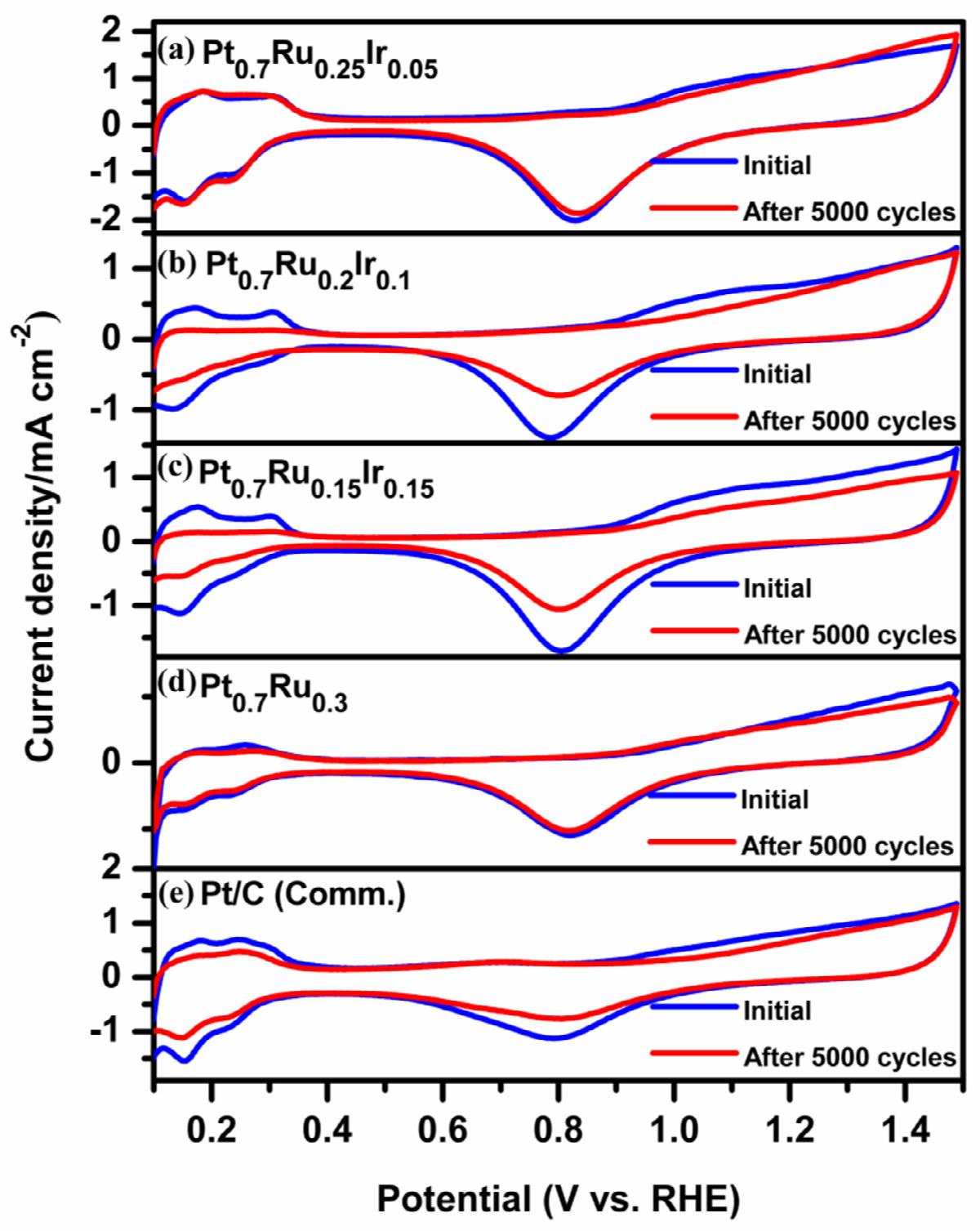
Fig. 8. ADT-cyclic voltammograms recorded in N2 saturated 0.5 M H2 SO4 electrolyte at 0.1 V
Pt0.7 Ru0.3 , and (e) Pt/C (Comm.) catalysts.
Figure 6. It clearly demonstrates that a trace amount of Ir might be more optimal for improved MOR activity. According to Sawy et al., increasing the Ir content in the catalyst composition weakens the bond of adsorbed CO and methanol to active Pt sites, causing the methanol adsorption/dehydrogenation reaction to slow down and the MOR activity to decrease even further [60].
From the respective CV profiles (Figure 5a), the order of If /Ib value was found to be in the following order: Pt0.7 Ru0.25 Ir0.05 (1.37) >Pt0.7 Ru0.15 Ir0.15 (1.35) >Pt0.7 Ru0.20 Ir0.10 (1.27) >Pt0.7 Ru0.3 (1.20) >Pt/C (Comm.) (1.14). The improved CO resistance of all Pt-Ru-Ir catalysts might be due to the synergistic effect between Pt, Ru, and Ir, as evidenced by the higher magnitude of If /Ib values during methanol oxidation, among other catalysts [19,56]. A Chronoamperometry (CA) test can also be used to assess the rate of CO poisoning during MOR and the catalysts’ stability at fixed potential overtime for all catalysts. Figure 7a depicts the CA profiles of all OMNs of Pt-Ru-Ir, Pt-Ru, and Pt/C (Comm.) catalysts recorded at 0.65 V for 3600 sec in a mixture of 0.5 M methanol + 0.5 M H2 SO4 solution [20,53,57].
The MOR oxidation currents of all catalysts were found to be decreasing steadily up to 500s and became constant up to 3600s. Based on the decay of oxidation current over time, the following formula can be used to calculate the percent of CO poisoning rate [22]:
where, j0 is the initial oxidation current density (mA cm 2 ), (dj/dt)t > 500s is the slope from the linear regression of current vs. time plot. The poisoning rate (δ ) for all three OMNs of Pt-Ru-Ir catalysts was found to between 0.0047 and 0.0056 % (Table 1), which is lower than Pt0.7 Ru0.3 (0.045%), standard Pt/C (Comm.) (0.044) catalysts. This strongly supports the high catalytic activity of Pt-RuIr catalysts attributed to the unique morphology and bifunctional mechanism induced by RuO2 and IrO2
The durability of electrocatalysts is critical in assessing their performance over time, which is investigated using the continuous potential cycling process, also known as the accelerated durability test (ADT). In Figure 8a-e, the CV profiles show the characteristics of all electrocatalysts before and after ADT, recorded at 0.1 V s 1 in N2 saturated 0.5 M H2 SO4 [41,59,61]. After 50 0 0 potential cycles, Pt0.7 Ru0.25 Ir0.05 is more stable than other catalysts, retaining nearly 80 % of its initial ECSA compared to Pt0.7 Ru0.2 Ir0.1 (68 %), Pt0.7 Ru0.15 Ir0.15 (62 %), Pt0.7 Ru0.3 (55 %), and Pt/C (Comm.) (60 %). The durability trend was exemplified from the bar chart of change in ECSA after 50 0 0 cycles of durability test for all three Pt-Ru-Ir, Pt0.7 Ru0.3 and Pt/C (Comm.) catalysts (Figure 7b). The extended stability was observed in terms of catalytic mass activity (Figure 9a-e) loss of nearly 48 % for Pt0.7 Ru0.25 Ir0.05 after 50 0 0 potential cycles in the evaluation of the MOR activity as performed under similar conditions of MOR-CV. As a result, the stability of OMNs of Pt-RuIr catalysts was found to be much better than that of Pt0.7 Ru0.3 and standard Pt/C (Comm.). This may be due to the support-free morphology with a key function of trace Ir content and particle
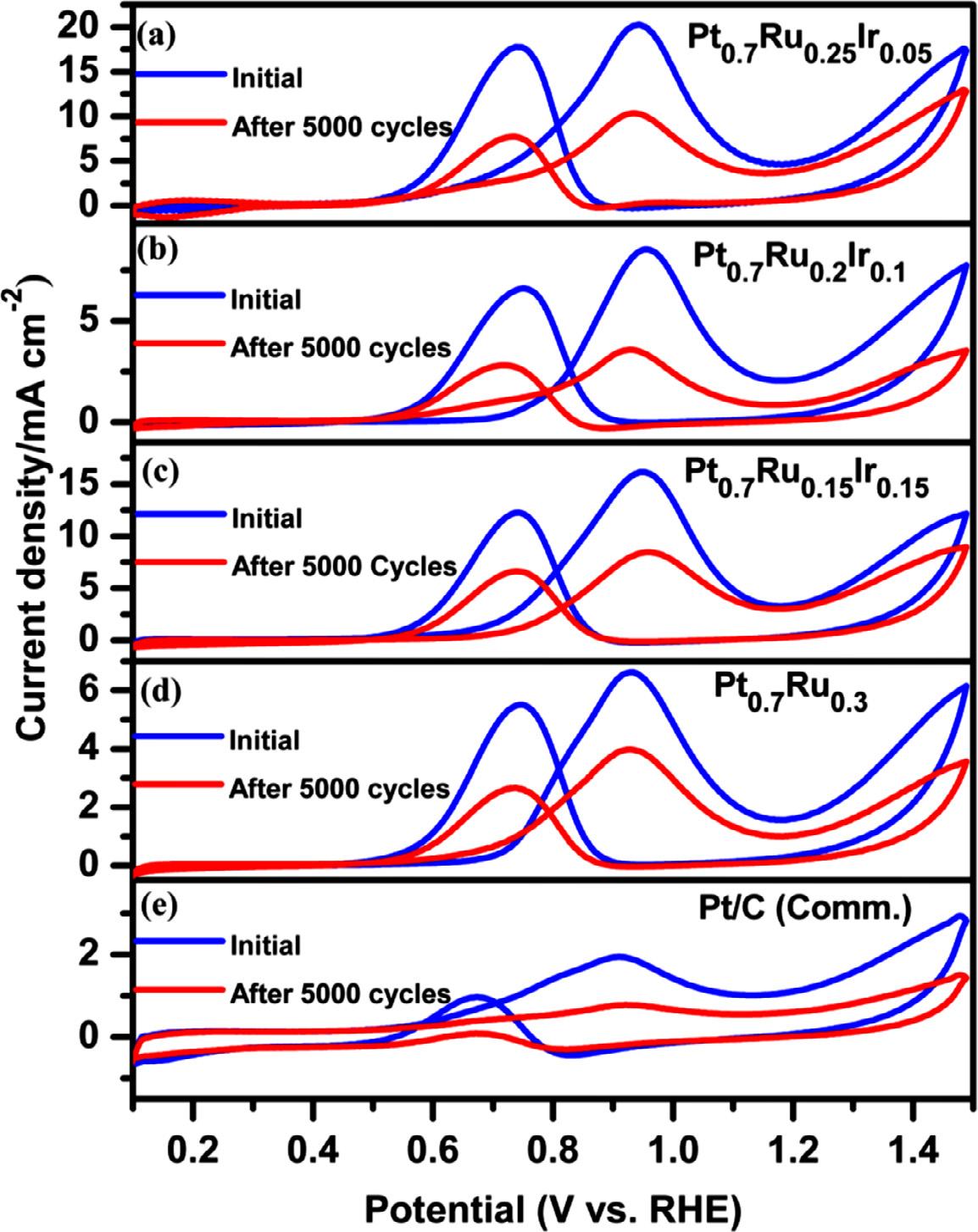
Fig. 9. ADT-cyclic voltammograms recorded in
, and (e) Pt/C (Comm.) catalysts.
size distribution, as well as a strong interaction between Pt, Ru, and Ir, as evidenced by the % of lattice compressive strain [22,47]. Notably, the Pt0.7 Ru0.25 Ir0.05 has outperformed stability and activity to the reported benchmark Pt-based multimetallic electrocatalysts for MOR (Table S2) [5,38,58]. Moreover, the alloy formation of Pt with Ru and trace amount of Ir, higher specific surface area, small crystallite and particle size of the Pt0.7 Ru0.25 Ir0.05 catalyst, as well as the co-existence of two critical components, Ruoxides and Ir-oxides, played key roles in the enhanced MOR activity and stability compared to other catalysts. The beneficial effect of the secondary metal Ru in Pt-Ru is attributed to a bifunctional mechanism, the activity was decreased by dissolution. As previously stated, the Ir content played a critical role in inducing the electronic effect with Ru by altering the electronic properties of Pt, as evidenced by the XRD and XPS results. Sawy et al. [60] demonstrated that the presence of oxophilic metals (Ir and Ru) with Pt have a significant impact on the bifunctional mechanism of MOR. According to their conclusions, increasing Ir content causes the downshift of Pt d-band center, resulting in weak interactions between surface adsorbents (such as methanol and CO) and active Pt sites [22,60,62,63]. More importantly, the strong electronic effect of Ir on Pt, depending on its composition, may weaken the methanol-Pt interaction, resulting in a lack of accessibility of active sites and sluggish methanol dehydrogenation, resulting in poor MOR activity and stability. Furthermore, the CA results demonstrated that the CO poisoning rate of Pt0.7 Ru0.25 Ir0.05 was relatively lower than that of other compositions and Pt0.7 Ru0.3 , Pt/C catalysts. This could be due to the fact that the trace amount of Ir has been well optimized based on the bifunctional effect on MOR as de-
scribed above. As a result, the synergism inferred from strong electronic effects with low Ir content, and lesser CO poisoning rate has been well evidenced in displaying the improved kinetics of MOR on Pt0.7 Ru0.25 Ir0.05 with extended stability.
4. Conclusion
In summary, OMNs of Pt-Ru-Ir catalysts with different elemental compositions were successfully prepared using the KIT-6 template-assisted chemical reduction process. TEM and XRD analysis were used to investigate the physicochemical properties of Pt-Ru-Ir catalysts, which showed that the Pt0.7 Ru0.25 Ir0.05 catalyst has an OMN morphology with a particle size distribution of around 4.51 nm and crystalline size of 3.59 nm. The mesoporous nature of the Pt0.7 Ru0.25 Ir0.05 catalyst, as well as the coexistence of RuO2 and IrO2 , were exposed by BET and XPS studies, which helps in promoting the electrocatalytic activity for MOR. As compared to Pt0.7 Ru0.3 and standard Pt/C (Comm.) catalysts, the Pt0.7 Ru0.25 Ir0.05 catalyst has a higher mass activity (1721 mA mg 1 ), ECSA (78.35 m2 g 1 ), and extended stability (retaining 80.5 % ECSA after 50 0 0 cycles), demonstrating the catalyst superior efficiency towards MOR. According to electrochemical studies, the presence of two active oxophilic metals (Ru and Ir) alloying with Pt, as well as the effect of trace Ir content, was determined and well correlated with its physicochemical features, taking into account the improved performance of Pt-Ru-Ir catalysts. The development of morphology-controlled trimetallic Pt-based nanostructures with rational secondary element compositions (Ru & Ir) has successfully demonstrated improved catalytic efficiency, and it might
S. Ravichandran, N. Bhuvanendran, Q. Xu et al.
be a promising strategy for designing potential anode materials for DMFC.
Declaration of Competing Interest
The authors declare that they have no known competing financial interests or personal relationships that could have appeared to influence the work reported in this paper.
Acknowledgements
We thank the financial support from National Key Research and Development Program of China (2018YFE0121200), National Natural Science Foundation of China (Nos. 21676126), China Postdoctoral Science Foundation (No. 2019M661751), Jiangsu Province Postdoctoral Fund (No. 2019K187) and the Priority Academic Program Development (PAPD) of Jiangsu Higher Education Institutions.
Supplementary materials
Supplementary material associated with this article can be found, in the online version, at doi:10.1016/j.electacta.2021.139148.
References
[1] Q. Liang, L. Zhang, M. Cai, Y. Li, K. Jiang, X. Zhang, P.K. Shen, Preparation and charaterization of Pt/functionalized graphene and its electrocatalysis for methanol oxidation, Electrochim. Acta 111 (2013) 275–283
[2] X. Wang, M. Sun, S. Xiang, M. Waqas, Y. Fan, J. Zhong, K. Huang, W. Chen, L. Liu, J. Yang, Template-free synthesis of platinum hollow-opened structures in deep-eutectic solvents and their enhanced performance for methanol electrooxidation, Electrochim. Acta 337 (2020) 135742
[3] B. Narayanamoorthy, K.K.R. Datta, M. Eswaramoorthy, S. Balaji, Highly Active and Stable Pt3Rh Nanoclusters as Supportless Electrocatalyst for Methanol Oxidation in Direct Methanol Fuel Cells, ACS Catal 4 (2014) 3621–3629
[4] X.L. Tian, L. Wang, P. Deng, Y. Chen, B.Y. Xia, Research advances in unsupported Pt-based catalysts for electrochemical methanol oxidation, J. Energy Chem. 26 (2017) 1067–1076
[5] S. Lu, K. Eid, D. Ge, J. Guo, L. Wang, H. Wang, H. Gu, One-pot synthesis of PtRu nanodendrites as efficient catalysts for methanol oxidation reaction, Nanoscale 9 (2017) 1033–1039.
[6] K. Deng, Y. Xu, D. Yang, X. Qian, Z. Dai, Z. Wang, X. Li, L. Wang, H. Wang, Pt–Ni–P nanocages with surface porosity as efficient bifunctional electrocatalysts for oxygen reduction and methanol oxidation, J. Mater. Chem. A 7 (2019) 9791–9797
[7] V. Thiagarajan, P. Karthikeyan, R. Manoharan, S. Sampath, A. Hernández-Ramírez, M.E. Sánchez-Castro, I.L. Alonso-Lemus, F.J. Rodríguez-Varela, Pt-Ru-NiTiO3 Nanoparticles Dispersed on Vulcan as High Performance Electrocatalysts for the Methanol Oxidation Reaction (MOR), Electrocatalysis 9 (2018) 582–592
[8] C. Li, T. Liu, T. He, B. Ni, Q. Yuan, X. Wang,Composition-driven shape evolution to Cu-rich PtCu octahedral alloy nanocrystals as superior bifunctional catalysts for methanol oxidation and oxygen reduction reaction, Nanoscale 10 (2018) 4670–4674
[9] Q. Wang, S. Chen, H. Lan, P. Li, X. Ping, S. Ibraheem, D. Long, Y. Duan, Z. Wei, Thermally driven interfacial diffusion synthesis of nitrogen-doped carbon confined trimetallic Pt3CoRu composites for the methanol oxidation reaction, J. Mater. Chem. A 7 (2019) 18143–18149
[10] X. Du, S. Luo, H. Du, M. Tang, X. Huang, P.K. Shen,Monodisperse and self-assembled Pt-Cu nanoparticles as an efficient electrocatalyst for the methanol oxidation reaction, J. Mater. Chem. A 4 (2016) 1579–1585
[11] S. Xue, W. Deng, F. Yang, J. Yang, I.S. Amiinu, D. He, H. Tang, S. Mu, Hexapod PtRuCu Nanocrystalline Alloy for Highly Efficient and Stable Methanol Oxidation, ACS Catal 8 (2018) 7578–7584
[12] L. Huang, Z. Wang, W. Gong, P.K. Shen, Atomic Platinum Skin under Synergy of Cobalt for Enhanced Methanol Oxidation Electrocatalysis, ACS Appl. Mater. Interfaces 10 (2018) 43716–43722.
[13] A.-X. Yin, X.-Q. Min, Y.-W. Zhang, C.-H. Yan, Shape-Selective Synthesis and Facet-Dependent Enhanced Electrocatalytic Activity and Durability of Monodisperse Sub-10 nm Pt Pd Tetrahedrons and Cubes, J. Am. Chem. Soc. 133 (2011) 3816–3819
[14] S. Sun, Q. Gao, H. Wang, J. Zhu, H. Guo, Influence of textural parameters on the catalytic behavior for CO oxidation over ordered mesoporous Co3O4, Appl. Catal. B 97 (2010) 284–291
[15] B.A. Kakade, T. Tamaki, H. Ohashi, T. Yamaguchi, Highly Active Bimetallic PdPt and CoPt Nanocrystals for Methanol Electro-oxidation, J. Phys. Chem. C 116 (2012) 7464–7470
[16] Y. Zhang, R. Shi, J. Ren, Y. Dai, Y. Yuan, Z. Wang, PtFeCu Concave Octahedron Nanocrystals as Electrocatalysts for the Methanol Oxidation Reaction, Langmuir 35 (2019) 16752–16760
[17] S. Yin, Z. Wang, X. Qian, D. Yang, Y. Xu, X. Li, L. Wang, H. Wang, PtM (M = Co, Ni) Mesoporous Nanotubes as Bifunctional Electrocatalysts for Oxygen Reduction and Methanol Oxidation, ACS Sustainable Chem. Eng. 7 (2019) 7960–7968
[18] Q. Wang, S. Chen, P. Li, S. Ibraheem, J. Li, J. Deng, Z. Wei, Surface Ru enriched structurally ordered intermetallic PtFe@PtRuFe core-shell nanostructure boosts methanol oxidation reaction catalysis, Appl. Catal. B 252 (2019) 120–127
[19] X. Bai, J. Geng, S. Zhao, H. Li, F. Li, Tunable Hollow Pt@Ru Dodecahedra via Galvanic Replacement for Efficient Methanol Oxidation, ACS Appl. Mater. Interfaces 12 (2020) 23046–23050
[20] M. Li, Y. Wang, J. Cai, Y. Li, Y. Liu, Y. Dong, S. Li, X. Yuan, X. Zhang, X. Dai, Surface sites assembled-strategy on Pt–Ru nanowires for accelerated methanol oxidation, Dalton Trans. 49 (2020) 13999–14008
[21] E.A. Franceschini, M.M. Bruno, F.J. Williams, F.A. Viva, H.R. Corti, High-Activity Mesoporous Pt/Ru Catalysts for Methanol Oxidation, ACS Appl. Mater. Interfaces 5 (2013) 10437–104 4 4
[22] N. Bhuvanendran, S. Ravichandran, W. Zhang, Q. Ma, Q. Xu, L. Khotseng, H. Su, Highly efficient methanol oxidation on durable PtxIr/MWCNT catalysts for direct methanol fuel cell applications, Int. J. Hydrogen Energy 45 (2020) 6447–6460
[23] P. Sivakumar, V. Tricoli, Novel Pt–Ru nanoparticles formed by vapour deposition as efficient electrocatalyst for methanol oxidation: Part II. Electrocatalytic activity, Electrochim. Acta 51 (2006) 1235–1243
[24] S. Kwon, D.J. Ham, T. Kim, Y. Kwon, S.G. Lee, M. Cho, Active Methanol Oxidation Reaction by Enhanced CO Tolerance on Bimetallic Pt/Ir Electrocatalysts Using Electronic and Bifunctional Effects, ACS Appl. Mater. Interfaces 10 (2018) 39581–39589
[25] H. Huang, J. Zhu, D. Li, C. Shen, M. Li, X. Zhang, Q. Jiang, J. Zhang, Y. Wu, Pt nanoparticles grown on 3D RuO2-modified graphene architectures for highly efficient methanol oxidation, J. Mater. Chem. A 5 (2017) 4560–4567
[26] W. Wang, Y. Li, H. Wang, Improved methanol oxidation on a PtRu–RuO2/C composite catalyst with close contact, Reaction Kinetics, Mechanisms and Catalysis 108 (2013) 433–441
[27] C. Zhou, H. Wang, J. Liang, F. Peng, H. Yu, J. Yang, Effects of RuO2 Content in Pt/RuO2/CNTs Nanocatalyst on the Electrocatalytic Oxidation Performance of Methanol, Chin, J. Catal. 29 (2008) 1093–1098
[28] H. Wang, S. Liu, H. Zhang, S. Yin, Y. Xu, X. Li, Z. Wang, L. Wang, Multinary PtPdNiP truncated octahedral mesoporous nanocages for enhanced methanol oxidation electrocatalysis, New J. Chem. 44 (2020) 15492–15497
[29] Z. Yan, L. Gao, C. Dai, M. Zhang, X. Lv, P.K. Shen, Metal-free mesoporous carbon with higher contents of active N and S codoping by template method for superior ORR efficiency to Pt/C, Int. J. Hydrogen Energy 43 (2018) 3705–3715.
[30] Q. Wang, Y. Zhang, Y. Zhou, Z. Zhang, J. Xue, Y. Xu, C. Zhang, X. Sheng, N. Kui, Nanocasting synthesis of an ordered mesoporous CeO2-supported Pt nanocatalyst with enhanced catalytic performance for the reduction of 4-nitrophenol, RSC Adv. 6 (2016) 730–739
[31] H. Huang, X. Hu, J. Zhang, N. Su, J. Cheng, Facile Fabrication of Platinum-Cobalt Alloy Nanoparticles with Enhanced Electrocatalytic Activity for a Methanol Oxidation Reaction, Sci Rep 7 (2017) 45555
[32] H. Huang, L. Ma, C.S. Tiwary, Q. Jiang, K. Yin, W. Zhou, P.M. Ajayan, Worm-Shape Pt Nanocrystals Grown on Nitrogen-Doped Low-Defect Graphene Sheets: Highly Efficient Electrocatalysts for Methanol Oxidation Reaction, Small 13 (2017) 1603013
[33] R. Jiang, D.T. Tran, J. Li, D. Chu, Ru@ RuO2 Core-Shell Nanorods: A Highly Active and Stable Bifunctional Catalyst for Oxygen Evolution and Hydrogen Evolution Reactions, Energy & Environmental Materials 2 (2019) 201–208
[34] N. Zhang, L. Bu, S. Guo, J. Guo, X. Huang, Screw Thread-Like Platinum–Copper Nanowires Bounded with High-Index Facets for Efficient Electrocatalysis, Nano Lett 16 (2016) 5037–5043
[35] H. Wang, H.Y. Jeong, M. Imura, L. Wang, L. Radhakrishnan, N. Fujita, T. Castle, O. Terasaki, Y. Yamauchi, Shape-and size-controlled synthesis in hard templates: sophisticated chemical reduction for mesoporous monocrystalline platinum nanoparticles, J. Am. Chem. Soc. 133 (2011) 14526–14529
[36] P. Karthika, H. Ataee-Esfahani, H. Wang, M.A. Francis, H. Abe, N. Rajalakshmi, K.S. Dhathathreyan, D. Arivuoli, Y. Yamauchi, Synthesis of Mesoporous Pt–Ru Alloy Particles with Uniform Sizes by Sophisticated Hard-Templating Method, Chemistry–An Asian Journal 8 (2013) 902–907
[37] H. Li, Y. Pan, D. Zhang, Y. Han, Z. Wang, Y. Qin, S. Lin, X. Wu, H. Zhao, J. Lai, B. Huang, L. Wang, Surface oxygen-mediated ultrathin PtRuM (Ni, Fe, and Co) nanowires boosting methanol oxidation reaction, J. Mater. Chem. A 8 (2020) 2323–2330
[38] L. Huang, M. Wei, N. Hu, P. Tsiakaras, P.Kang Shen, Molybdenum-modified and vertex-reinforced quaternary hexapod nano-skeletons as efficient electrocatalysts for methanol oxidation and oxygen reduction reaction, Appl. Catal. B 258 (2019) 117974
[39] T. Audichon, T.W. Napporn, C. Canaff, C.u. Morais, C.m. Comminges, K.B. Kokoh, IrO2 coated on RuO2 as efficient and stable electroactive nanocatalysts for electrochemical water splitting, J. Phys. Chem. C 120 (2016) 2562–2573
[40] Y. Lee, J. Suntivich, K.J. May, E.E. Perry, Y. Shao-Horn, Synthesis and activities of rutile IrO2 and RuO2 nanoparticles for oxygen evolution in acid and alkaline solutions, J. Phys. Chem. Lett. 3 (2012) 399–404
[41] K. Peng, N. Bhuvanendran, S. Ravichandran, W. Zhang, Q. Ma, Q. Xu, L. Xing, L. Khotseng, H. Su, Bimetallic Pt3Mn nanowire network structures with enhanced electrocatalytic performance for methanol oxidation, Int. J. Hydrogen Energy 45 (2020) 30455–30462
[42] Y. Qin, M. Luo, Y. Sun, C. Li, B. Huang, Y. Yang, Y. Li, L. Wang, S. Guo, Intermetallic hcp-PtBi/fcc-Pt Core/Shell Nanoplates Enable Efficient Bifunctional
S. Ravichandran, N. Bhuvanendran, Q. Xu et al.
Oxygen Reduction and Methanol Oxidation Electrocatalysis, ACS Catal 8 (2018) 5581–5590
[43] Q. Wang, S. Chen, J. Jiang, J. Liu, J. Deng, X. Ping, Z. Wei, Manipulating the surface composition of Pt–Ru bimetallic nanoparticles to control the methanol oxidation reaction pathway, Chem. Commun. 56 (2020) 2419–2422
[44] R. Bavand, A. Korinek, G.A. Botton, A. Yelon, E. Sacher, PtRu Alloy Nanoparticles I. Physicochemical Characterizations of Structures Formed as a Function of the Type of Deposition and Their Evolutions on Annealing, J. Phys. Chem. C 121 (2017) 23104–23119
[45] J. Xie, Q. Zhang, L. Gu, S. Xu, P. Wang, J. Liu, Y. Ding, Y.F. Yao, C. Nan, M. Zhao, Y. You, Z. Zou, Ruthenium–platinum core–shell nanocatalysts with substantially enhanced activity and durability towards methanol oxidation, Nano Energy 21 (2016) 247–257
[46] J. Yi, W.H. Lee, C.H. Choi, Y. Lee, K.S. Park, B.K. Min, Y.J. Hwang, H.-S. Oh, Effect of Pt introduced on Ru-based electrocatalyst for oxygen evolution activity and stability, Electrochem. Commun. 104 (2019) 106469.
[47] V. Baglio, R.S. Amin, K.M. El-Khatib, S. Siracusano, C. D’Urso, A.S. Aricò, IrO2 as a promoter of Pt–Ru for methanol electro-oxidation, PCCP 16 (2014) 10414–10418
[48] D. Geng, D. Matsuki, J. Wang, T. Kawaguchi, W. Sugimoto, Y. Takasu,Activity and Durability of Ternary PtRuIr C for Methanol Electro-oxidation, J. Electrochem. Soc. 156 (2009) B397
[49] J. Park, H.J. Kim, A. Oh, T. Kwon, H. Baik, S.-I. Choi, K. Lee, RuOx-decorated multimetallic hetero-nanocages as highly efficient electrocatalysts toward the methanol oxidation reaction, Nanoscale 10 (2018) 21178–21185
[50] L. Huang, X. Zhang, Q. Wang, Y. Han, Y. Fang, S. Dong, Shape-Control of Pt–Ru Nanocrystals: Tuning Surface Structure for Enhanced Electrocatalytic Methanol Oxidation, J. Am. Chem. Soc. 140 (2018) 1142–1147
[51] G. Chen, X. Yang, Z. Xie, F. Zhao, Z. Zhou, Q. Yuan, Hollow PtCu octahedral nanoalloys: Efficient bifunctional electrocatalysts towards oxygen reduction reaction and methanol oxidation reaction by regulating near-surface composition, J. Colloid Interface Sci. 562 (2020) 244–251
[52] S. Lu, K. Eid, M. Lin, L. Wang, H. Wang, H. Gu, Hydrogen gas-assisted synthesis of worm-like PtMo wavy nanowires as efficient catalysts for the methanol oxidation reaction, J. Mater. Chem. A 4 (2016) 10508–10513
[53] M. Li, Q. Jiang, M. Yan, Y. Wei, J. Zong, J. Zhang, Y. Wu, H. Huang, Three-dimensional boron-and nitrogen-codoped graphene aerogel-supported Pt nanoparticles as highly active electrocatalysts for methanol oxidation reaction, ACS Sustainable Chem. Eng. 6 (2018) 6644–6653
[54] C. Yang, Q. Jiang, W. Li, H. He, L. Yang, Z. Lu, H. Huang, Ultrafine Pt Nanoparticle-Decorated 3D Hybrid Architectures Built from Reduced Graphene Oxide and MXene Nanosheets for Methanol Oxidation, Chem. Mater. 31 (2019) 9277–9287
[55] M.S. Khalifeh-Soltani, E. Shams, E. Sharifi, Pt–Ru nanoparticles anchored on poly(brilliant cresyl blue) as a new polymeric support: Application as an efficient electrocatalyst in methanol oxidation reaction, Int. J. Hydrogen Energy 45 (2020) 849–860
[56] C. Li, Y. Qu, L. Du, G. Chen, S. Lou, Y. Gao, G. Yin, Synthesis of Well-Defined Pt-Based Catalysts for Methanol Oxidation Reaction Based on Electron–Hole Separation Effects, ACS Sustainable Chem. Eng. 7 (2019) 8597–8603
[57] Q.-X. Chen, C.-X. Yu, H.-H. Li, Z. He, J.-W. Liu, Composition Modulation of Pt-Based Nanowire Electrocatalysts Enhances Methanol Oxidation Performance, Inorg. Chem. 59 (2020) 1376–1382
[58] W. Chen, Z. Lei, T. Zeng, L. Wang, N. Cheng, Y. Tan, S. Mu, Structurally ordered PtSn intermetallic nanoparticles supported on ATO for efficient methanol oxidation reaction, Nanoscale 11 (2019) 19895–19902.
[59] W. Xiong, B.A.T. Mehrabadi, S.G. Karakolos, R.D. White, A. Shakouri, P. Kasak, S.J. Zaidi, J.W. Weidner, J.R. Regalbuto, H. Colon-Mercado, J.R. Monnier, Enhanced Performance of Oxygen-Functionalized Multiwalled Carbon Nanotubes as Support for Pt and Pt–Ru Bimetallic Catalysts for Methanol Electrooxidation, ACS Appl. Energy Mater. 3 (2020) 5487–5496
[60] E.N. El Sawy, V.I. Birss, Understanding the role of Ir during methanol oxidation at PtxIry alloy nanoparticles, J. Electrochem. Soc. 164 (2017) F1572
[61] Z. Bai, J. Luo, D. Ming, C. Wang, H. Xu, W. Ye, High active and durable N-doped carbon spheres-supported flowerlike PtPd nanoparticles for electrochemical oxidation of liquid alcohols, Electrochim. Acta 356 (2020) 136794
[62] K. Eguiluz, G. Salazar-Banda, D. Miwa, S. Machado, L. Avaca, Effect of the catalyst composition in the Ptx (Ru–Ir) 1 x/C system on the electro-oxidation of methanol in acid media, J. Power Sources 179 (2008) 42–49
[63] N. Bhuvanendran, S. Ravichandran, S.S. Jayaseelan, Q. Xu, L. Khotseng, H. Su, Improved bi-functional oxygen electrocatalytic performance of Pt–Ir alloy nanoparticles embedded on MWCNT with Pt-enriched surfaces, Energy 211 (2020) 118695