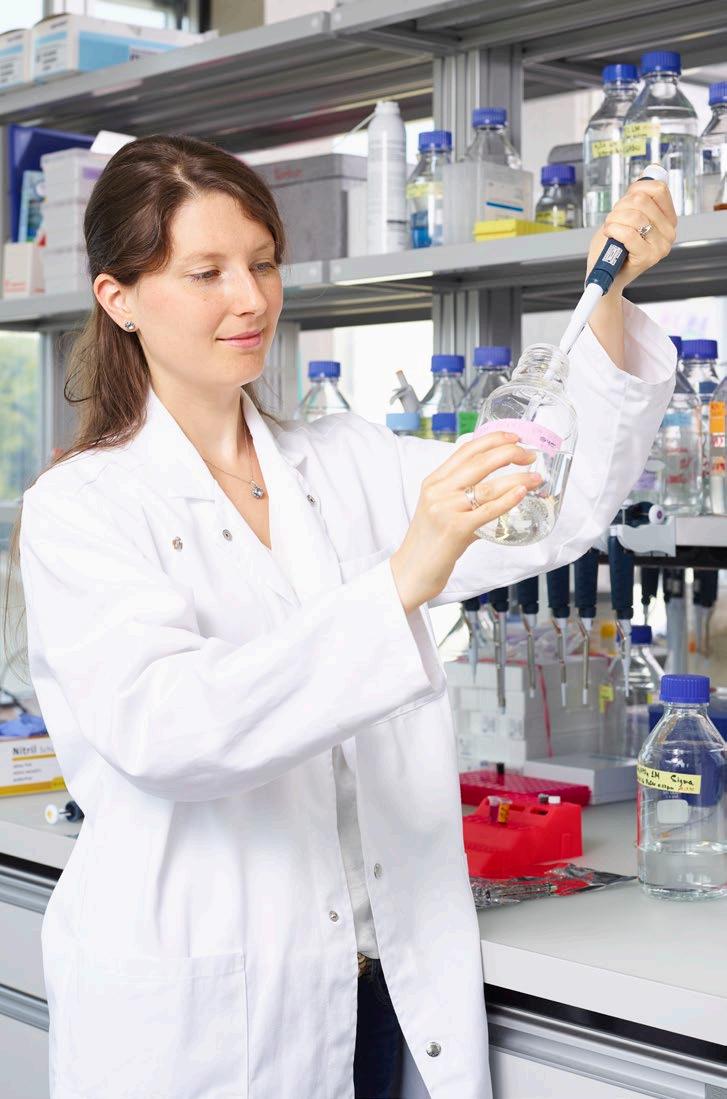
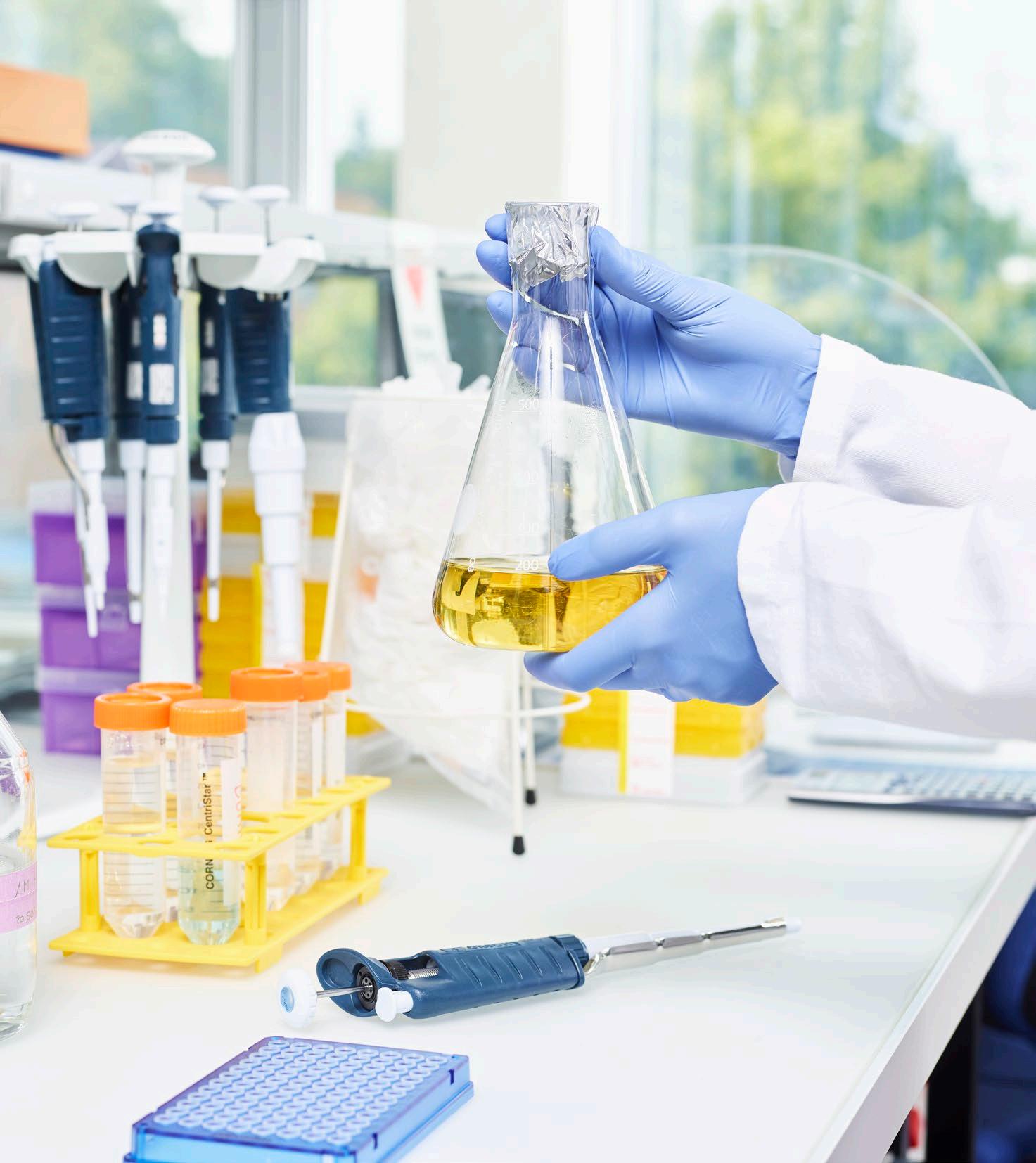
When researchers in pharmaceutical companies develop new drugs nowadays, they rely on detailed scientific findings about the causes behind particular diseases. PSI contributes to this knowledge in multiple ways. For one thing, PSI researchers investigate fundamental structures of life and, on that basis, develop approaches for new treatment methods. In addition, they develop radiopharmaceuticals – radioactive drugs with which certain tumours can be localised and precisely destroyed.
At the same time, the large research facilities of PSI, which are unique in Switzerland, are also open to researchers from universities and industrial enterprises who come here to study structures of vital biomolecules and medicinal agents. Thus there is a bit of PSI in many thirdparty research results and therapies.
Beyond that, there are patients who profit directly from the work of PSI: people who are afflicted by certain kinds of cancer and are treated with the precise and gentle proton therapy right on the campus of the Institute.
In the fundamental research of PSI, one of the essential classes of basic biological building blocks plays the leading role: proteins, highly complex biomolecules that control and carry out practically all metabolic processes as well as cell division in all forms of life. In humans and animals they also form the support scaffolding of the cell and transport materials within the cell.
Again and again, PSI researchers gain notice in expert circles for describing proteins in an unprecedented degree of detail and thus elucidate their functionality. Among the proteins studied most intensively at PSI are those that control the process of vision as well as cell division.
A second research focus at PSI is on the development of radiopharmaceuticals. These drugs, which for the most part consist of a combination of a biomolecule that accumulates on tumour tissue with a radioactive isotope, are used in hospitals for cancer diagnostics and therapy.
Still, PSI’s contribution to the understanding of life processes extends far beyond its own research: Numerous external experts in the areas of biology and biochemistry carry out experiments at the large research facilities of PSI. Among them are also, besides scientists from universities, researchers from large pharmaceutical firms or emerging biotech companies – some of which have been founded directly out of PSI. Through the interplay between their own research and their ingenuity in engineering, PSI experts advance the development of these facilities in such a way as to ensure future progress in medicine. Thus the new X-ray laser SwissFEL makes it possible not only to record static images of proteins, but also to measure the ultrafast changes in protein molecules after their activation and, in this way, to make a film
about the protein activation – and do it with a resolution at which the individual atoms of the protein can be seen.
Proton therapy shows how fundamental research in other fields can contribute to advances in medicine in astonishing ways. When this treatment method was developed in the 1980s, PSI was the only institution in Switzerland that possessed a suitable proton accelerator, which had been built for physics research. Today hundreds of patients per year are treated here, including very many children. In addition, PSI is a leader in the further development of this innovative treatment method.
On the following pages you can learn more about the secrets of life that are elucidated at PSI, as well as the usefulness of this research for forward-looking applications in medicine.
Since 1984, more than 8,000 cancer patients, including 500 children, have been treated by means of the ultraprecise proton therapy.
The share of PSI’s budget devoted to life sciences amounts to 24 percent.
Since 2002, more than 5,600 protein structures have been deciphered at SLS.
Studies at the large research facilities contribute to our knowledge about the structures of life and establish the basis for new drugs.
Proteins are central building blocks of every living system. Like tiny, highly specialised machines, they perform countless tasks in the living organism: They break down materials and build up others, form the support scaffolding of our cells, transmit information within the body, and see to it that energy is stored up and made available again on demand. Although they are all assembled according to the same principle – from a chain of “standardised” building blocks, the amino acids – they can have an almost unimaginable variety of different forms. At PSI, researchers decipher the structure and function of the most diverse proteins and thus gain a better understanding of life processes. With this knowledge, they contribute to the development of new drugs.
Practically all processes in our body are regulated by proteins. These complex biomolecules can be thought of as tiny machines that take on the most diverse tasks in the cells of our body. They make it possible for us to see, hear, and taste, and they take care that different areas of the body can communicate and coordinate with each other. All nutrients such as sugars, fats, and vitamins are assembled or metabolised by proteins, as are likewise all cellular components including DNA, RNA, and proteins themselves. Proteins also play a role in all diseases: For example, people can become ill when their bodies’ proteins are malformed or when the organism is attacked by microorganisms, which again consist to a large extent of proteins. Therefore it is little
wonder that medicinal treatment in most cases is aimed at proteins. Each protein consists of a chain of smaller molecules – the amino acids. The arrangement of these amino acids for every single protein is stored in the DNA. But the order of the amino acids isn’t the only thing that matters. It is also important that this chain folds correctly and in doing so forms its typical three-dimensional structure, with various extensions and bulges. In addition, practically all proteins alter their shape when they do their work – so they have more than one structure each. Only when scientists know the three-dimensional structure of the protein as well as how it changes can they really understand its function.
At PSI, several research groups are investigating the composition and function of proteins. One area of focus is the so-called G protein-coupled receptors – proteins that sit in the cell membrane and see to it that information from the outside gets into the cell. A familiar example of such a protein is the beta receptor of the heart muscle – if molecules of the hormone adrenalin from outside come into contact with such a receptor, they stimulate the muscle cells to beat more strongly. To artificially reduce this effect, the receptors can be blocked with the help of a drug – a beta blocker. Overall, 30 percent of all newly developed drugs attack proteins of this family. Structural biologists at PSI have determined the
The receptor rhodopsin has seven spiral structures. It is located in the cell membrane (yellow background). Visible between the spirals, with a shape like a panicle of grapes, is the retinal, which reacts to light. The incidence of light causes the retinal to change its form and thus slightly shift the spirals. This allows a G protein (beige-grey structure) from inside the cell (turquoise background) to bind to the rhodopsin receptor. The G protein initiates a process that makes sure the information of the light signal is passed on to the nerve cells and finally to the brain. This process takes place constantly and at such a fast pace that our eyes can perceive changes in the environment instantaneously.
light-induced structural changes in another G protein-coupled receptor, the visual pigment rhodopsin. This receptor reacts to light and is responsible for the “translation” of the physical information “light” into a biochemical signal that can be transmitted to the nerve cells in the brain. The PSI researchers are working to elucidate the exact details of this process on the molecular
level; among other things, the insights gained yield important information for the development of new drugs against diseases that cause blindness.
Another example is the protein tubulin. It forms thin fibres that take on various functions in the cells. The fibres serve as tracks on which substances are transported within the cell, and they form an important part of the “skele-
ton” that holds the cell in shape and coordinates the machinery of cell division. During cell division, tubulin fibres pull apart the newly formed chromosomes and distribute them between the two new cells. The latter proves useful in chemotherapy, in that this process can be interrupted with agents that bind to tubulin and thus prevent the division of cancer cells. The eluci-
dation of the structure of tubulins in interaction with different agents at PSI enables the improvement or new development of active ingredients for chemotherapy. In addition, the tubulins are the basis for tiny, highly mobile hairs that enable locomotion in single-celled organisms and can also be found on certain tissue surfaces, as in the lungs, for example, where they transport mucus outwards.
Proteins are also crucial for the development of radiopharmaceuticals –these cancer drugs dock selectively on proteins on the surface of tumour cells and destroy them with their radiation. The research facilities of PSI, which are also open to researchers from all over the world as well as industrial enterprises, offer in part unique possibilities for determining the structure and function of proteins. Members of staff at PSI look after the external users during their experiments, and thanks to this experience they are able to constantly enhance the facilities.
The central role proteins play for the effectiveness of numerous drugs also accounts for their economic significance. In the development of new drugs, many large pharmaceutical companies make use of insights into the structure of proteins that they have obtained at PSI. The protein research environment at PSI has also generated a series of spin-off enterprises, which for example develop active medicinal agents or support industrial users in their own investigations at PSI.
Gebhard Schertler is the head of the research division of Biology and Chemistry at the Paul Scherrer Institute PSI and Professor for Structural Biology at ETH Zürich. In this interview he talks about biological research at PSI and the future of drug development
Gebhard Schertler, one of the core competences of PSI is deciphering the structure of proteins. What makes proteins so interesting?
In biology, proteins make up the workbench of life. They are structural building blocks or active tools that transform materials or transmit signals. Therefore, they are central to understanding the essential processes of life. There’s a saying: “The language of life is chemistry.” And a great many proteins are catalysts, which enable or regulate this chemistry.
What is the significance of protein research for our everyday life?
Fundamental biology is becoming ever more relevant for the development of new therapeutic options against diseases. Thus, the insights we are gaining about the structure of proteins help to develop better drugs – for example, against cancer or diseases of the eye. But there is even more potential: We are standing at the start of a technological revolution, which could be described as biological systems engineering. Proteins become components in new biological regulatory circuits. For example, today people are thinking about drugs that can be switched on or off with light. At the moment this is possible only to a limited extent, but it will likely become
very important in the future; projects at PSI are providing the necessary research infrastructure for these developments.
The buzzword “personalised medicine” keeps coming up in connection with the future of diagnostics and therapy. What is meant by this term?
Personalised medicine has different aspects. On the one hand, it refers to a treatment that is tailored to one particular person – that can begin with a genetic test, or with the medical history and imaging techniques: for instance, when precisely defining, in the course of proton therapy at PSI, the three-dimensional zone around the tumour that should be treated. On the other hand, you want to analyse a person’s genes and environmental factors as completely as possible and thus find out, for example, whether cancer cells will respond to a particular hormone therapy or not. For me, the essence of it is that in the end, after a precise analysis of the individual patient, an optimal treatment emerges.
And how are researchers at PSI contributing to personalised medicine?
In our fundamental research, we are concerned with how proteins interact with each other. Through analysis we try to determine which protein plays a central role in a disease: This protein is then called the target molecule, because it becomes the attack point for the medical treatment. The researchers here at PSI often play a very important role when such a target molecule has already been identified and subsequently needs to be investigated more precisely, whether structurally, biophysically, or kinetically.
The results of these studies then yield clues as to which drugs are most suitable for the treatment.
In this context, what does “rational” drug design mean – and what would be the alternative?
In the past, the approach was to try to investigate thousands and thousands of chemical compounds in one big testing procedure – in the hope of identifying, by chance, a single compound that showed the desired effect. Yet this “non-rational” approach didn’t stand the test. Millions were invested – and in the end, the successes were small. Therefore, today, there is really no longer any alternative to rational drug design where, on the basis of a target molecule, a suitable active ingredient is developed. With this approach we have had good experience with biological agents, for example with antibodies. Once a target molecule has been rationally identified as the cause of a disease, it can be switched on or off with antibodies. There are disadvantages of antibodies in that they generally need to be injected, and may activate undesired immunological reactions. Therefore, most pharmaceutical researchers are always searching for soluble substances that can be taken in tablet form. Here, too, there are major advances – on the one hand through structural biology, on the other through computer modelling of proteins. It’s a combination of practical experience and theory that pays off best in the end.
In what other areas is PSI developing personalised medicine?
Above all, radiopharmacy should be mentioned here, where radiopharma-
ceuticals, radioactively marked active ingredients , are being developed in close collaboration with hospitals. These are used mostly in the diagnosis of cancers, but also increasingly in therapy.
Beyond that, what strategies do you follow within the life sciences research at PSI? Is there a vision that guides the way?
Strategically, we are at the moment strongly focused on making a contribution to personalised medicine. At the same time, besides fundamental questions in structural biology, we will give priority to topics that have relevance for the pharmaceutical industry, and we will intensify our collaboration with hospitals. It is important to understand that the way we gain knowledge is changing. Up to now it was possible to achieve major breakthroughs with individual experimental methods alone. In the future it will become ever more important to find combinations of methods that are best suited to solve a specific problem and to make new findings possible. Therefore, we invest in a broad portfolio of methods. For one, PSI has recently built the free-electron X-ray laser SwissFEL, with which the movement of proteins can be made visible in the form of, one might say, ‘molecular movies’. On another front, structural analysis by means of electron beams is becoming increasingly important – here, too, we are working on new machines. One important goal for the future is to determine the structure of proteins in their natural environment, inside cells.
They are sometimes the last hope against cancer: radioactive agents that find their own way to the tumour cells and fight the cancer inside the body. Other “radiating medicines” have proven their value as diagnostic agents: In a PET scanner, for instance, they can indicate if the brain is functioning properly. At PSI, more than 25 researchers are engaged with radiopharmaceuticals – they develop new agents or produce them for use in hospitals.
Although it’s impossible to imagine modern medicine without them, in the public perception they lead a shadow existence: radiopharmaceuticals. These are medicines that incorporate a radioactive substance, which decays in the body and emits radiation. Radiopharmaceuticals can be used in two ways: Either the radioactive substance delivers a lot of energy in a narrowly defined area, making it suitable for destroying a tumour, or they emit radiation only weakly
but over a long range. Then their signals can be detected by sensitive measurement devices outside the body, thus providing information about structures and processes in the organism. In most cases a complex biomolecule that aims selectively for a particular target molecule on tumour cells serves as the carrier for the radioactive substance. The target molecules are usually proteins, so the work in radiopharmaceuticals also fits in with the protein research at PSI.
Radiopharmaceuticals are studied, refined, and even produced at PSI. Five work groups take part in interdisciplinary collaborations in the Centre for Radiopharmaceutical Sciences, which PSI manages jointly with ETH Zurich and the University Hospital Zurich. Physical, chemical, biological, pharmaceutical, and medical aspects must be considered in equal measure when the goal is to produce a suitable drug for the correct application – timed just right and matched with the individual patient. When working with radioactivity, high safety standards must be met. Furthermore, the short shelf life of the drugs due to radioactive decay must be taken into account.
It can take many years before a drug has been developed to the point where it can be used in clinical studies. One successful example is 177Lu-PSIG-2. That’s the name given to a new PSI-developed radiopharmaceutical that is directed at a special cancer of the thyroid and its metastases. The cancer arises from the so-called C cells of the thyroid – radio-iodine therapy does not help in this case. Since the end of 2016, the new preparation has been tested at the University Hospital of Basel within the framework of a clinical study. It consists of a small protein that resembles one of the body’s own hormones together with the radioactive substance lutetium-177. The small protein docks selectively on receptors on the surface of the tumour cells. The radioactive substance, the so-called radionuclide, decays there and destroys the cancer in a targeted manner. As simple as this is in principle, however, the pathway to the drug was dif-
ficult. Among the challenges were the initial side-effects of the hormone-like protein, the accumulation of the radiopharmaceutical in healthy tissue, and the necessity of optimising the production method.
The approach of another research group at PSI is still a dream for the future: Their goal is to channel radioactive drugs directly into the nucleus of a cancer cell. The radioactive substance could then make a direct strike on the genetic material and thus prevent further divisions of the cancer cell. This would be similar in principle to the usual chemotherapy to date, only much more selective and with many fewer side-effects. With terbium-161, the researchers have already found a suitable radionuclide. Its radiation has an extremely short range and results in a high energy release. Now the radionuclide still needs to be coupled with a suitable protein that can open the way for it through the nuclear envelope of the cell.
A radionuclide with completely different characteristics has been sought after by radiochemists at PSI, to improve the availability of radiopharmaceuticals for so-called positron-emission tomography or PET. With a PET scanner, three-dimensional images of the interior of the body can be produced, and biochemical processes in the organism can be made visible. Besides diagnosing tumours and metastases, it can be used for example to detect pathological changes in the brain.
For one thing, to be suitable for a PET scanner, the sought-after radionuclide must emit a specific kind of particle –positrons. Also, it should decay more slowly than the radionuclides currently being used. That’s because their extremely short decay time restricts the distribution of the drugs, even within a country as small as Switzerland. So the PSI scientists are now testing a new candidate that satisfies both requirements: scandium-44. They produce the radioactive material by bombarding non-radioactive calcium-44 with protons for 90 minutes. For that they use a particle accelerator at PSI. Thus the special infrastructure of the research institute may be opening the path for a new radionuclide to serve all of the hospitals in Switzerland using a PET scanner.
For the development of new radiopharmaceuticals, PSI has built up a laboratory infrastructure that is unique in the canton of Aargau and beyond. With that, it also meets the requirements for being permitted to produce the radiopharmaceutical 68Ga-PSMA, developed elsewhere, which it recently began supplying to the Aargau Canton Hospitals in Baden and Aarau. With the help of this agent, doctors can diagnose metastases of prostate cancer early and initiate treatment in a timely way. Because of the short half-life of the isotope employed, gallium-68, 68GaPSMA is only usable for three hours and must therefore be produced near where it will be applied. With this work PSI is helping to bypass a significant bottleneck in the drug supply in Aargau.
Only through teamwork can the complex experiments to determine protein structures at SLS be carried out.
At the large research facilities of PSI, scientists make structures of biomolecules and biological tissues visible. With that, they help to better understand processes in organisms and establish the basis for the development of new drugs. Physicists and engineers work constantly to improve the measurement methods so that researchers can investigate ever more challenging questions.
Proteins are highly complex biomolecules that are central for all of the processes in the human organism. For the development of modern drugs, it is often crucial to understand the structure of the proteins involved, yet these are only a few millionths of a millimetre in size and therefore too small to be observable with a light microscope. At present a strong source of X-ray light is usually required in order to decipher their structure.
In the experiment, X-ray light shines through the proteins – that is, the light goes in at one place and comes back out again at another. Along the way, part of the light is diverted from its straight path. These diffractions are registered by a detector. From this information, with the help of sophisticated computer programs, the structure of the proteins can be determined. It is not possible to examine one individual biomolecule, though – what’s needed is a protein crystal in which many identical biomolecules are arranged in a regular three-dimensional pattern. The production of such crystals is an art in itself, which researchers in a special laboratory at PSI have perfected.
The X-ray light for such experiments can only be generated at complex large research facilities. PSI operates two such facilities. The Swiss Light Source SLS went into operation at PSI in 2001. Here, at three measurement stations, researchers can determine the struc-
ture of protein molecules in detail. At the end of 2016, the X-ray free-electron laser SwissFEL was inaugurated – here researchers can observe how protein molecules change their shape, thus gaining a better understanding of their function. SwissFEL represents a novel type of research facility – currently, there are only four other facilities of its kind worldwide.
The basic principle behind SwissFEL and SLS is similar: Both take advantage of the fact that fast electrons emit light when they are forced onto a curved path. In both facilities, a particle accelerator first brings the electrons up to nearly the speed of light. In SLS, the accelerator is roughly circular and has a circumference of 288 metres; in SwissFEL, a linear accelerator around 300 metres long is used. A regular arrangement of many magnets then forces the electrons onto a slalom track, thus causing them to generate light. The characteristics of the light depend on the details of the facility –so SLS mostly generates a quasi-con-
tinuous beam of light, while SwissFEL emits short flashes of extremely intense light.
Among the 5,600 proteins whose structure has been deciphered at SLS since it started operating are structures of the visual pigment rhodopsin, which functions as a light sensor in our eyes. Also the structure of the ribosome, a large and very complex biomolecule, which in the cell is responsible for the assembly of other proteins, was resolved in part with help from measurements at SLS. For this achievement, the Cambridge-based structural biologist Venkatraman Ramakrishnan received the 2009 Nobel Prize in Chemistry.
To always keep up with the growing demands of the scientists, PSI continually enhances the measurement equipment. So, for example, they work to make the PSI-developed detectors that register the rays diffracted upon penetrating protein crystals ever faster and more accurate.
To be able to observe proteins in motion at SwissFEL, researchers at PSI have refined the method of serial crystallography and are leading participants in the development of so-called time-resolved serial crystallography. Many small crystals of the same protein are X-rayed one after another with the extremely short and intense light pulses of SwissFEL. Shortly before the measurement, the protein is activated with light at slightly different points in time. In each crystal, then, the protein is arranged in a different phase of the motion. When they are put together, the images generated in the process yield the desired “film”.
In this way, an international team under the leadership of PSI researchers was able to produce a 3-D film in 2018 that revealed important insights into one of the fastest processes in biology. The researchers were able to show how different components of bacteriorhodopsin, a molecule activated by light, similarly to the human visual pigment, work together to make the process especially efficient. Since SwissFEL was not yet ready at the time of the experiment, the measurements were carried out at a comparable facility in the USA. Protein structures are not the only topic from the life sciences studied at the large research facilities at PSI. The composition and functionality of cellular components, biological tissues and whole organs, and of drugs as well, can be investigated at SLS or the Swiss Spallation Neutron Source SINQ.
To ensure that the large research facilities can achieve their full potential, researchers develop and test ancillary devices that are employed at the separate experiment stations.
Physicist Oliver Bunk, head of the Laboratory for Macromolecules and Bioimaging at the Paul Scherrer Institute, explains why so many researchers from the life sciences prefer to come to PSI to conduct experiments.
methods are used at PSI to make biological structures visible?
Mr. Bunk, your area of expertise is called “macromolecules and bioimaging”. Could you please explain what
Far more than one method. In the life sciences, interesting phenomena occur on every level: That begins with atoms, which join together to form complex molecules, and extends to visible phenomena such as hand or mouth movements. One important method, on the level of large molecules, is X-ray crystallography. It yields the structures of proteins, which take part in all of the
processes of life. It is significant not only for fundamental research, but also for the pharmaceutical industry. And on a larger scale, it is possible here to look at tissue samples, or to conduct experiments on living mice that contribute to understanding and minimising the harmful effects of artificial respiration on premature babies.
At SLS, you image molecules with intense Xray light. That requires re
searchers from the life sciences – including for example biologists, physicians, and pharmaceutical scientists – to collaborate closely with physicists and engineers. How is this cooperation organised at PSI? In my team at SLS, we have a lot of physicists, because they’re good at building instruments. But for the application, they then need to talk with colleagues who are experts in the respective fields about their research questions and install special technical systems: Biological samples, for example, often need to be cooled to prevent radiation damage. Or the samples have to be kept moist. Mutual exchanges across disciplinary boundaries are necessary to build the best experiments. Normally from our side there are mixed teams of physicists and biologists on site at the so-called beamline. They are experts in the examination method, and they have built the experiment. As part of PSI’s service for users, they work together closely with researchers who have requested beamtime to resolve their own questions, whether they come from PSI or from outside. And together they tackle the experiment.
How would you characterise the researchers who use SLS for experiments in the life sciences? What fields do these users come from? What kinds of questions do they bring with them?
Many have specialised in structural biology and come from academia –from ETH Zurich and the EPF Lausanne, for example, but also half from universities outside Switzerland – as well as from the pharmaceutical industry. For these groups, X-ray crystallography is an important part of their research, and they know a lot about the technology. But we also have exciting projects in the area of neuroscience, for example concerning Alzheimer’s and Parkin-
son’s diseases. Here many of the researchers don’t know anything about the possibilities of the X-ray method. But the first pioneers are coming to us – or also scientists whom we’ve made aware of the new methods.
What special capabilities does PSI offer its external users – for instance in comparison to similar facilities worldwide? Why do researchers choose PSI? At SLS we don’t offer all methods, but what we do, we try to do very well – we focus more on quality than quantity. We’re not just good in instrument development; we also provide good, collaborative user supervision. Often in the Anglo-American regions, only technical support is offered; the research is left to the users. By contrast we recruit researchers for the User Office who already have experience with the facilities from their own experiments, who are now optimising these, and who would like to cooperate with the best researchers in the world for the advancement of science. They are highly self-motivated. One can’t help noticing that very good scientific publications come out of this – more than in comparable facilities. High quality – that’s just the Swiss approach.
Beamtime at the large research facilities of PSI is limited, but in great demand. How is it decided who, in the end, will get to use the facilities?
We operate the beamlines 5,000 hours per year, 220 days per year. When we are in operation, we work six days a week, around the clock. Then once the experiments have started, they should not be interrupted; otherwise you would have a lot of start-up time. And these are expensive machines that must be utilised as fully as possible. Nonetheless, we have considerably more demand than we can satisfy. So
who can measure, and when, has to be regulated. We organise that through an international committee. Two times per year, academic users can submit proposals. The have to describe, on two to three pages, what experiment they plan to do. The committee, which consists of independent subject-matter experts, reviews and ranks the proposals. Then the best are granted beamtime at our facility.
Academic users are not required to pay anything. In return, they must publish the results afterwards, so that they are freely available to the scientific community. Industrial users who want to keep the results to themselves have to pay. One hour of beamtime costs around 1,000 Swiss francs. Large pharmaceutical companies book large allotments of time with us at a somewhat reduced rate.
Could you please explain, for example, how an experiment at SLS is typically prepared, and how it then proceeds?
From the idea to the publication, two years can easily go by. When researchers have an idea for which some alteration of the facility is anticipated, a preliminary discussion takes place on site. Otherwise they simply write their proposals and, in successful cases, receive an allotment of beamtime within the next half-year – together with a contact on site who can be consulted by phone in the run-up to the experiment. Then the experiment is carried out – that can last between eight hours and an entire week, depending on the technique and how demanding the experiment is. Then comes the data analysis. Often additional measurements or laboratory studies are needed before the results can be published.
Determining the composition and function of protein molecules is the goal of most researchers who study the structures of life at the large research facilities of PSI. Yet the laboratories of PSI offer numerous other possibilities for investigations in the life sciences. With Xray light, for example, it is also possible to capture 3D images of tissue structures and make the processes in organs visible. Neutrons reveal the composition of thinner organic layers. That could someday help to ensure that the body receives the correct dosage of drugs.
Doctors and medical researchers have long wished for a method that would give them three-dimensional images of tissue structures with an accuracy down to a few nanometres. This could be used to image, in samples of brain tissue for example, clumps of proteins such as are typical for Parkinson’s and other degenerative diseases. Here studies using modern microscopes yield information that is definitely important, yet the samples have to be prepared in a way that falsifies the structures. To examine samples with a light microscope, for example, they must be dyed; only very thin slices of tissue can be examined with an electron microscope.
In recent years scientists at PSI have developed and perfected a method with which they can produce detailed images of three-dimensional structures in a deep-frozen cube of tissue with an edge length of roughly one-tenth of a millimetre. In the process, X-rays from SLS illuminate the sample at many
different points. From the results of many individual measurements, a sophisticated computer program determines the three-dimensional structure of the tissue being examined. Besides the special X-ray beam, the technical requirements include for example a mechanism for moving and positioning the sample with nanometre precision, a chamber in which the tissue can be deep-frozen before and during the measurement, and the software that generates the 3-D images. The new platform, known by the acronym OMNY, enables the examination of various types of tissue and thus can also be used, for example, for cancer research. These methods have already shown their strengths in studies of bone structures, which have yielded evidence for research in osteoporosis, as well as in investigations of brain samples that enable new insights for the neurosciences, in particular for Parkinson’s research.
When children are born prematurely, their lungs are not yet mature. They must then receive artificial respiration in the incubation chamber. But it is not easy, for example, for neonatal medical staff to set the air pressure of the respirator correctly. With the pressure too low or too high, there is a risk of lasting damage to the lungs. Therefore scientists are searching for methods that can simulate artificial respiration in animal experiments. They would like to be able to observe the tiny alveoli of laboratory mice live and in motion.
At one beamline of SLS that is specialised for generating detailed 3-D images of various materials, the first successful attempts to observe the alveoli have now been made. While recording the heartbeat with an EKG, the scientists managed to consistently trigger each shot with a flash of X-ray light when the heart was in a relatively relaxed state, so that the image of the lungs would not be blurry. The X-ray light has to act within just one to two milliseconds, or else the image is fuzzy.
In the future, in vivo microscopy is expected to help optimise artificial respiration, but that’s not all. It should also clear up fundamental questions in lung physiology that have long been waiting for answers. The same goes for the question of what goes wrong on the level of the alveoli in diseases such as asthma and pulmonary fibrosis. In this work PSI’s cooperation partners are the clinic for neonatology at Lausanne University Hospital as well as institutes of ETH Zurich and the University of Bern.
Not only X-rays but also neutron beams are employed at PSI for life science experiments. The facility used for that
purpose is the Swiss Spallation Neutron Source SINQ at PSI, which otherwise mainly helps materials scientists inspect the atomic structure of materials. Food and nutrition researchers at ETH Zurich have questions to clarify for which optical examination methods are inadequate. They want to know more about the characteristics of biopolymers that form a thin film on water –only one to two molecular layers thick. How such films behave at different temperatures, at different degrees of acidity, and under the influence of digestive enzymes has already been investigated at SINQ. Cellulose is one example of such a biopolymer. Researchers want to shape this roughage material in such a way that drugs can be microencapsulated in it, so that they only release their active agent at body temperature. Another goal is, by putting biopolymers into fatty foods, to ensure that they are not digested in the stomach, but only when they reach the small intestine. That way people would feel full more quickly and ingest less fat overall.
In another project, PSI researchers are developing and building a novel apparatus that will make it possible for the first time to observe the structure of biological molecules in their natural setting – inside the living cell. This is a new kind of electron microscope that will employ electron beams to examine molecular structures.
Irradiation with protons is an especially conservative way to attack certain tumours. In close collaboration with specialised clinics, the Centre for Proton Therapy CPT at PSI has been offering this cancer therapy since 1984. More than 8,000 people, including more than 500 children, have been treated here since then. Alongside the treatment of patients, an important focus at CPT is on investigating and continually refining treatment methods.
More than half of all cancers are curable today, and many patients owe their survival to radiation therapy. Besides the classical radiation therapy with X-rays or gamma rays, there is treatment with particle beams – often with beams of protons. They have the property that they hit the tumour very precisely and thus protect surrounding healthy tissue considerably better than other radiation therapies. For physical reasons, protons release their maximal energy very precisely at a predetermined point in the tissue.
That in Switzerland proton therapy is based not at a hospital, but rather at PSI, is due to the long-standing experience here with generating beams of fast protons. Since 1974, a proton accelerator facility has been in operation at PSI that is used for experiments in physics and the materials sciences. It was also, in the beginning, the one that provided the beam for the treatment of patients. Since 2007, there is COMET, an accelerator especially optimised for cancer therapy, which was developed by a company together with PSI researchers.
In 1984, patients affected by an ocular tumour began to receive treatment with protons at PSI. Today a good 200 patients with ocular tumours are still being treated at PSI annually. Since the end of the 1990s, the so-called spot-scanning technique, which was developed at PSI, has strongly expanded the possibilities of proton therapy. This new method enables the exact
treatment of tumours that are located deep within the body and difficult to reach, such as brain tumours, for example, as well as tumours in the areas of the head, neck, pelvis, and spine. Children in particular benefit from this form of proton therapy: Their organs are very sensitive to radiation, and their long life expectancy makes it important to minimise the risk of side-effects from the irradiation.
In spot scanning – also called pencil beam scanning – a highly focused pro-
ton beam, which at a thickness of five to seven millimetres is about as thin as a pencil, scans the entire volume of the tumour, point by point and layer by layer. Thus there is hardly any strain on the tissue surrounding the tumour. Crucial for this is the technology for steering the proton beam, which is housed in an installation weighing around 200 tons – the gantry. With millimetre precision, this colossal machine can be rotated around the patients. For more than a decade, this
method was available only at PSI. In the meantime it has become the worldwide standard in proton therapy. Before proton treatment begins, the position and extent of the tumour must be precisely determined. Also, patients
are not permitted to move during the irradiation. Adults are aided in this by an individual face mask, a dental night guard, or a couch that fits the body exactly. Small children are given light anaesthesia, so that they lie quite still for the duration of the irradiation.
Alongside the treatment of patients, the researchers of CPT work to refine the method through a number of different projects. They are making proton therapy even safer and more efficient, and they take great care to make the treatment less stressful for patients. In addition, they are developing new technologies that should expand the range of applications for spot-scanning proton therapy. In the future, one new irradiation technique is expected to allow tumours in organs that are by nature always in motion – for example, the lungs with their airways – to be irradiated with protons.
A great many experts work at CPT. Various teams concern themselves with service and maintenance for the highly specialised therapy facilities. Regular quality checks and inspections by the Federal Office of Public Health ensure its smooth and safe operation. The medical staff is not only specialised in the exacting treatment, but also in caring support for the patients. Close collaboration exists with special clinics throughout Switzerland – in patient care as well as in research.
Not only does PSI operate the only treatment centre for proton therapy in Switzerland, but PSI researchers have also done considerable work to advance the technology behind the method.
Before protein molecules can be examined at SLS, they have to be incorporated into crystals. Producing such crystals is an art that researchers in a special laboratory of PSI have perfected.
One technique that was developed for experiments at SLS is expected, in the future, to enable mammography examinations that distinguish between diseased and healthy tissue better than the current method.
The fundamentals underlying most new drugs or medical devices originate in publicly funded research institutions such as PSI. For example, pharmaceutical companies investigate active ingredients at SLS or use the understanding of life processes obtained at PSI for the development of new drugs. Other companies benefit from PSI in that they utilise and build on technology and knowhow developed at PSI.
users just send in their samples and, at the end, receive a report with a detailed interpretation of the findings.
oped and produced at PSI. The gap between two “lattice bars” is just a few thousandths of a millimetre.
Someone being treated, for example, with a new drug for cancer may not realise that a large research centre such as PSI might have taken part in its development. And yet that’s the case: Practically all large pharmaceutical firms in the world, and quite a few smaller ones as well, use the Swiss Light Source SLS to find new active agents or to optimise known remedies. That’s because under the focused X-ray beam, their interaction with the body’s own proteins can be studied with precision. This tight collaboration between PSI and the pharmaceutical industry will also continue at the X-ray laser SwissFEL. Here it will be possible to follow the changes in the molecules during such an interaction and thus obtain further important information for the optimisation of the agent. All industrial clients bear the costs for use of the facilities themselves. The Swiss companies Roche and Novartis, which have been present since 2002, even co-finance one of the three SLS beamlines designed for protein studies. In carrying out, evaluating, and interpreting the measurement results, pharmaceutical researchers from industry are supported by PSI researchers or by two specialised PSI spin-offs. This assistance extends so far that some
Researchers at PSI investigate the molecular backgrounds of a range of diseases and determine which specific classes of substances could be used to treat these diseases. With this fundamental research, they establish the basis for drug development, which can then be pursued by the pharmaceutical companies. For example, a cooperation between PSI and Roche builds on PSI research into hereditary degenerative eye diseases. Within the framework of this cooperation, the researchers have developed a method with which candidate agents for the treatment of these diseases can be identified. The goal is to develop drugs that slow down the progression of the disease and thus help patients maintain their vision longer.
SLS has also inspired medical research in the area of cancer prevention: PSI physicists discovered, shortly after the turn of this century, how an examination method that had previously been used only at the large research facility can also be carried over to standard X-ray systems. This so-called phase-contrast X-ray technique employs properties of X-ray light that are disregarded in conventional X-ray examinations. It makes it possible to clearly demarcate not only hard structures such as bones, but also a variety of soft tissues of the body. The X-ray beam must be guided through several very fine lattices, which were devel-
In 2011, in collaboration with the Canton Hospital of Baden, researchers succeeded in proving that the method is suitable for mammography, that is, X-ray examination of the female breast. Further clinical studies have shown that the new method promises a better way to discriminate between benign and malignant changes in the breast. Together with the electronics concern Philips, the research group has now built an initial prototype for a mammography device suitable for application in hospitals and doctors’ practices.
The newest irradiation faciity, Gantry 3, was built in a research collaboration with the firm Varian Medical Systems. The long-standing teamwork between the industry partners with their knowhow and PSI, with its expert knowledge stemming from fundamental and applied research for proton therapy, has been a major learning experience for both partners.
In 1993 the firm Schaer Proton AG, based in Flaach in the canton of Zurich, worked with PSI to build the world’s first treatment facility (Gantry 1) using the PSI-developed spot-scanning technique for proton therapy. For several years, Schaer has been manufacturing almost exclusively systems and components for proton therapy and marketing these worldwide.
Thus the work of PSI benefits companies in Switzerland and people facing illnesses all over the world.
Numerous discoveries that have been made at PSI in the field of life sciences have the potential to form the basis for new drugs or medical technologies. In some cases, the researchers responsible then decide to found a spinoff – a company that further develops the results, translates them to practice, and brings them to market. Other PSI spinoffs relieve industrial users of substantial amounts of the work involved in carrying out their experiments at the large research facilities of PSI. PSI actively promotes the founding of spinoff companies by its researchers.
The firm InterAx combines experimental and computer-aided pharmacology to support biotech and pharmaceutical companies in the development and selection of substances that could be used as new drugs in the future. The technologies used rest on the foundation of research results obtained by Martin Ostermaier and Aurélien Rizk – two of the company’s founders – in the course of their work at PSI. The researchers of InterAx first investigate experimentally how the interaction of a potential drug molecule with the target molecule proceeds over time. With the help of the experimental findings, they can simulate the effect of the agent with the help of specially developed computer programs. These simulations provide insights that are important for the development of the agent but which cannot be obtained using present-day experimental methods. Under the leadership of the three
company founders Martin Ostermaier, Luca Zenone and Aurélien Rizk as well as the research head Maria Waldhoer, InterAx has developed into a dynamic start-up that has aroused the interest of Swiss and international investors. One of the first companies to have opened its offices in Park innovaare, the innovation park in the immediate vicinity of PSI, in 2015, is leadXpro. The leadXpro team develops new, custom-designed agents for drugs that are expected to significantly improve therapy for diseases such as, for example, cancer and infectious diseases. In doing so, leadXpro especially brings in its staff members’ long years of experience in two areas: the investigation of G protein-coupled receptors, ion channels, and so-called transporters; and the use of the large research facilities of PSI, such as the Swiss Light Source SLS and the X-ray free-electron laser SwissFEL. Currently leadXpro is working on joint projects with six industrial partners from Europe and the USA and in addition is developing its own agent pipeline.
Araris is among the latest spin-offs from PSI. It concentrates on establishing a novel manufacturing method that enables the optimisation, development, and production of high-potency medicines: in particular, so-called antibody-drug conjugates. These consist of an antibody – a complex biomolecule that docks on a particular target molecule with extreme selectivity – and the
actual medicinal agent. Thus the antibodies can selectively transport the agent to the diseased cell. With the antibody-drug conjugates produced by Araris, diseases such as cancer or inflammatory diseases and bacterial infections can be fought more effectively and with less side-effects in the future. On his way to becoming a businessman, Araris founder Philip Spycher was supported by a PSI Founder Fellowship. This new funding instrument provides an 18-month grant that supports young researchers and engineers of PSI on the career path to entrepreneurship, not only financially but also through coaching and consultation. Within this period the grantees must demonstrate the commercialisation potential of their business ideas and prepare an initial business plan.
In July 2017, the start-up GratXray was founded. Its goal: Develop, manufacture, and commercialise devices that offer – thanks to phase-contrast technology (see pages 26-27) – a method for diagnosing breast cancer that is more both precise and less stressful for the patient than the mammography technique used today. In the same year it was founded, the firm was honoured with the Swiss Technology Award.
Numerous pharmaceutical companies investigate drugs or protein structures at SLS. Two specialised PSI spin-offs support them in these studies – they conduct the experiment and if required deliver a detailed report with the findings.
have taken on the job of supporting researchers from industry in their studies at SLS.
Expose, which was founded in 2008 by PSI researcher Joachim Diez, assists industrial researchers who want to conduct studies to elucidate protein structures. The scientists from Expose take over all tasks involved in the measurement. For Swiss pharmaceutical companies, this means that they can get the measurement results they need in the shortest amount of time and thus more rapidly advance the development of new drugs. Companies from more distant regions, on the other hand, can have their samples studied at SLS with-
out needing to send their own staff members to Switzerland. If required, the researchers at Expose also evaluate the measurement results and provide the customer with complete information about the structure of the molecule under investigation. Expose employs four people and is currently collaborating with 19 industrial partners at 27 locations in Europe, North America, and Asia.
Excelsus, which was founded by PSI researcher Fabia Gozzo in 2012, offers pharmaceutical companies studies at SLS using the powder diffractometry method. This method makes it possible, among other things, to determine
the arrangement of active ingredient molecules in drugs. That is important because an undesirable arrangement can have dangerous consequences and must be avoided at all costs. It can lead to a faster than expected release of the drug, in which case the patient can rapidly receive a dose that is much too high. Excelsus staff members act as scientific partners of the colleagues from the pharmaceutical companies: They carry out the measurements at SLS, evaluate the results, and often suggest solutions for their research questions. Excelsus employs five people. In May 2016, the company opened offices in Park innovaare.
The circular building in the background is the Swiss Light Source SLS, on the west bank of the River Aare. The SwissFEL X-ray free-electron laser is located in the forest across the river, on the left of the picture.
The Paul Scherrer Institute PSI is a research institute for natural and engineering sciences, conducting cutting-edge research in the fields of future technologies, energy and climate, health innovation and fundamentals of nature. By performing fundamental and applied research, we work on sustainable solutions for major challenges facing society, science and economy. PSI develops, constructs and operates complex large research facilities. Every year more than 2500 guest scientists from Switzerland and around the world come to us. Just like PSI’s own researchers, they use our unique facilities to carry out experiments that are not possible anywhere else. PSI is committed to the training of future generations. Therefore about one quarter of our staff are post-docs, post-graduates or apprentices. Altogether PSI employs 2200 people, thus being the largest research institute in Switzerland.
Concept/Text
Judith Rauch/Dr. Paul Piwnicki
Editing committee
Dr. Paul Piwnicki, Christian Heid
Photography and Graphics
All Photos Scanderbeg Sauer
Photography except:
page 9: Dr. Ching-Ju Tsai
page 18: Kellenberger Kaminski
Photographie
page 20: Dr. Sarah Shahmoradian
page 30: Markus Fischer
Design and Layout
Monika Blétry
Printing
Paul Scherrer Institut
Available from Paul Scherrer Institut
Events and Marketing
Forschungsstrasse 111
5232 Villigen PSI, Schweiz
Tel. +41 56 310 21 11
Villigen PSI, February 2019
Head of Research Division
Biology and Chemistry
Prof. Dr. Gebhard Schertler
Tel. +41 56 310 42 65 gebhard.schertler@psi.ch
Head of the Macromolecules and Bioimaging Laboratory
Dr. Oliver Bunk
Tel. +41 56 310 30 77 oliver.bunk@psi.ch
Head of the Centre for Proton Therapy
Prof. Dr. Damien C. Weber
Tel. +41 56 310 58 28 damien.weber@psi.ch
Head of the Centre for Radiopharmaceutical Sciences
Prof. Dr. Roger Schibli
Tel: +41 56 310 28 37 roger.schibli@psi.ch
Contact for industrial companies that would like to conduct investigations at the large research facilities:
CEO SLS Techno Trans AG
Stefan Müller
Tel. +41 56 310 54 27 stefan.mueller@psi.ch
Head Corporate Communications
Dr. Mirjam van Daalen
Tel. +41 56 310 56 74 mirjam.vandaalen@psi.ch